Structures and stability of the Cu38 cluster at finite temperature
Abstract
The UV-visible and IR properties of the Cu38 nanocluster depend to a great extent on the temperature. Density functional theory and nanothermodynamics can be combined to compute the geometrical optimization of isomers and their spectroscopic properties in an approximate manner. In this article, we investigate entropy-driven isomer distributions of Cu38 clusters and the effect of temperature on their UV-visible and IR spectra. An extensive, systematic global search is performed on the potential and free energy surfaces of Cu38 using a two-stage strategy to identify the lowest-energy structure and its low-energy neighbors. The effects of temperature on the UV and IR spectra are considered via Boltzmann probability. The computed UV-visible and IR spectrum of each isomer is multiplied by its corresponding Boltzmann weight at finite temperature. Then, they are summed together to produce a final temperature-dependent, Boltzmann-weighted UV-visible and IR spectrum. Additionally, Molecular Dynamics simulation of the Cu38 nanocluster was performed to gain insight into the system dynamics and make a three-dimensional movie of the system with atomistic resolution. Our results show the thermal populations at the absolute temperature of Cu38 cluster, and the disordered structure that dominates at high temperatures.
pacs:
61.46.-w,65.40.gd,65.,65.80.-g,67.25.bd,71.15.-m,71.15.Mb,74.20.Pq,74.25.Bt,74.25.Gz,74.25.KcI Introduction
Nano clusters are of interest due they allow us to study the transition from free atoms to bulk condensed systemsB517312B as a consequence, analyze the size-dependent evolution of their properties.doi:10.1021/cr040090g Especially, Noble-Metal Nanoclusters (NMC) have attracted attention in many fields of science due to interesting plasmonic, catalytic properties,Inwati2018 ; https://doi.org/10.1002/ppsc.201400033 and photophysical properties at nanoscale,Xavier2012 Particularly, Nano Cu clusters embedded in the dielectric matrix have attracted attention because of their tunable longitudinal surface plasmon resonance.Inwati2018 Besides, copper is cheaper than gold and silver, and it has large photosensitivity, high thermal and electric conductivity, and optical properties Zhang2019 that makes it a good candidate to develop nanodevices C5RA14933A and nanoelectronics. Jena10560 In particular, Cu38 attracted attention due to it has a magic structures, B912501A defined in terms of geometric and energetic factors and related to the closing of electronic shells PhysRevB.69.235421 as it happens in small sodium clusters.RevModPhys.65.611 For the Cu38 cluster its magicity is due to only energetic considerations.A709249K ; PhysRevB.69.235421 In contrast, small packed barium clusters with magic numbers, the stability is dominated for geometrical effects rather than electronic effects.RevModPhys.65.611 It is believed that magic structures are the putative global minimum energy structures on the potential energy surface, thus reflect the molecular properties of the system.PhysRevB.69.235421 From the experimental point of view, the Cu38 cluster has been widely studied by photoelectron spectroscopy techinque (PES). Pettiette et al.doi:10.1063/1.454575 employing PES extract the electronic gap of anionic Cu38 cluster and found a semiconductor type with 0.33 eV.doi:10.1063/1.454575 ; Zhang2019 However, the geometrical structure was not investigated. In contrast, Kostko et al. also studied the anionic Cu38 and from the PES inferred that putative global minimum should be an oblate structure instead of a high symmetric structure,Kostko2005 ; Zhang2019 despite that computations for 38 atom clusters on noble metal clusters frequently found high symmetric (cuboctahedral) structures.A709249K ; Fujima1989 ; Kostko2005 From the theoretical point of view, Taylor et al. presented a study based on density functional theory (DFT) of thermodynamic properties of Cu38 cluster,Taylor_2008 Prevoius works employing DFT studied the transition states and reaction energies of water gas shift reaction in a Cu38 cluster and Cu slab.doi:10.1142/S021963362050008X In other previously DFT studies the high symmetry octahedral structure was reported as the lowest energy structuredoi:10.1021/acs.jpcc.6b13086 employing PW91Vosko functional, plane wave basis set and pseudopotential approximation.doi:10.1063/1.3187934 Hijazi et al. Hijazi2010 investigated the Cu38 cluster employing hybrid strategy; they used the embedded atom method potential followed by DFT computations using the PBE functional and pseudopotential approximation and reported that octahedral (OH) symmetry is the putative global minimum structure followed by the incomplete-Mackay icosahedron (IMI) located at 0.26 eV above of the putative global minimum. On the other hand, search for the lowest energy structure employing many body potentials gives a cuboctahedral structure.A709249K ; Grigoryan2005 Erkoc et al. PhysRevA.60.3053 employing an empirical potential-energy function, which contains two-body atomic interactions Erkoc1994 found that the fivefold symmetry appears as putative global minimum in Cu38 cluster. In contrast, the cuboctahedron structure was reported as putative global minimum in a previous studiesPhysRevB.73.115415 employing empirical many-body Gupta and Sutton-Chen potentials. Nevertheless, there has been some discussion which is the lowest energy structure, some previous works consider the Cu38 octahedron cluster, as the putative global minimumHijazi2010 ; doi:10.1021/acs.jpcc.6b13086 ; doi:10.1063/1.3187934 , in contrast, several others found that Cu38 cluster with the truncated octahedron geometry is energetically more stable than other configurationsZHAO2017111 ; Hijazi2010 ; doi:10.1063/1.3187934 ; doi:10.1021/acs.jpcc.5b05023 ; Darby ; jp1048088 ; doi:10.1080/08927022.2011.616502 . We point out that the energy computed with different methods such as DFT, MP2, or CCSDT, just to mention few of them, yield different energetic ordering.Buelna ; molecules26185710 ; Puente In the case of DFT, the functional and basis set employed, ZPE energy correction, or energy of dispersion among others can interchange the putative global minimum.
Moreover, practical molecular systems and materials needs warm temperature PhysRevLett.79.1337 , so the molecular properties at temperatures T are dominated by Boltzmann distributions of isomers,Buelna ; MENDOZAWILSON2020112912 ; molecules26133953 therefore, their properties are statistical averages over the ensemble of conformations.Buelna The structure corresponding to the global minimum ceases to be the most likely at high T so other structures prevail. Furthermore, in small Ag clusters, the temperature leads the transition from the initial FCC phase to other structural modifications,Redel2015 so it promotes the changes of fases in materials. Interesting, at different temperatures than zero, the molecular system minimizes the Gibbs free energy and maximizes the entropy.Buelna ; molecules26133953
Although the search of the global and local minima is useful in understanding reactivities and catalytic efficiencies, but such studies most of the time neglect temperature dependent entropic contributions to free energy when increasing temperature. Taking temperature into account requires dealing with nanothermodyanamics.Buelna ; molecules26133953 ; Hill ; Li-Truhlar ; Truhlar ; Baletto ; Grigoryan ; Bixon The thermodynamics of clusters have been studied by a variety of tools,calvo ; Wales925 ; Buelna ; Li-Truhlar ; Truhlar like molecular-dynamics simulations on boron clusters Gerardo and Cu38 clusters.Zhang2019 The cluster properties depends strongly of the structure, size, composition, and temperature, so the first step in order to understanding molecular properties is the elucidation of the lowest energy structure and its isomers close in energy; molecules26133953 ; Buelna ; Baletto ; Darby ; Ohno ; Buelna2021a This is a complicated task due to several factors.Buelna ; molecules26133953 As second step for understanding cluster properties relies on their spectroscopy which gives insight into its structure and it was proposed as a way of detecting structural transformations into clusters. The influence of temperature on the spectroscopy has been computed before for a variety of clusters, for instance, Uzi ; Buelna ; molecules26133953 such in the present study, for the neutral Cu38 cluster, we use the statistical formulation of thermodynamics or nanothermodynamicsLi-Truhlar ; Buelna ; Truhlar ; molecules26133953 to compute thermodynamics properties and define the putative global minimum at temperatures diferent from zero, evaluated the relative populations among the isomers and computed UV-Visible and IR spectra as a Boltzmann weighted spectrum sum of individual spectra. Our findings show that at hot temperatures at amorphous structure strongly dominate the putative global minimum whereas the truncated octahedron dominates at cold temperatures. The remainder of the manuscript is organized as follows: Section 2 gives the computational details and a brief overview of the theory and the algorithms used. The results and discussion are presented in section 3; The putative global at room temperature and relative population in ranging temperatures from 20 to 1500K, and the IR spectra as a function of temperature. Conclusions are given in Section 4.
II Theoretical Methods
II.1 Method to Explore the Free Energy Surface and Computational Details
At temperatures different of zero, the Gibbs free energy determines the lowest-energy structure,10.3389/fchem.2022.841964 whereas at temperature zero the enthalpy determines the putative global minimum.Buelna ; molecules26133953 A simple analysis of the Gibbs free energy given by deals to a conclussion, in order to minimize the Gibbs free energy we must to maximize the entropy.Buelna ; molecules26133953 ; 10.3389/fchem.2020.00757 Front the theoretical point of view, and first of all, with the aim to understand molecular properties, at temperatures non-zero, we must know the lowest Gibbs free energy structure or the largest entropy structure, and all structures closest in energy to the lowest energy structureBuelna ; molecules26133953 or all high-entropy structures closet in entropy to the largest entropy structure. We must keep in mind that the experiment are performed at non-zero or finite temperatures. From a very general point of view, it has been shown that the validity of DFT can be extended to finite temperatures by the concept of ensemble DFT.10.3389/fchem.2019.00106 The search of the global minimum in atomic clusters is complicated task due to the number of possible combinations grows exponentially with the number of atoms leading to a combinatorial explosion problem among others.Buelna ; molecules26133953 Despite that is not an easy task, several algorithms to explore globally the potential/free energy surface coupled to a local optimizer generally of any electronic structure package have been successfully employed in a target so far, i.e. AIRSS approach,Pickard_2011 simulated annealing,kirkpatrick ; metropolis ; xiang ; yang ; vlachos ; granville kick methodology,Sudip ; Cui ; Vargas-Caamal2 ; Vargas-Caamal ; Cui2 ; Vargas-Caamal2015 ; Florez ; Ravell ; Hadad ; Saunders ; Saunders2 ; Grande-Aztatzi and genetic algorithms Guo ; Dong ; Mondal ; Ravell ; Grande-Aztatzi ; Kessler ; Alexandrova ; Buelna among many others. Global structure searching at the DFT level are computationally expansive to be applicable to intermediate and large clusters size, as we mentioned early, the number of candidates increase exponentially. In this work we used two-stage procedure to explore efficiently the potential energy surface, in the first stage we perform a global search using a empirical methodology, where Gupta interaction potential were used to describe the Cu-Cu interactions with default parameters taken from referencesB204069G ; PhysRevB.48.22 and coupled to Basin Hopping global optimization algorithm implemented in Python code and part of global search of GALGOSON code.Buelna ; molecules26133953 At the second stage, all the lowest energy structures from the first stage are symmetrized, followed by a DFT optimization that were performed using the Gaussian suite codegauss employing two exchange-correlation functionals, B3PW91, PBE and two basis set, def2SVP and LANL2DZ, with and without taking into account D3 version of Grimme’s dispersion correctionsGrimme as implemented in Gaussian 09 code.gauss The Becke’s hybrid three-parameterdoi:10.1063/1.464913 ; PhysRevA.38.3098 exchange-correlation functional in combination with the Perdew and Wang GGA functional PW91 PhysRevB.45.13244 ; Vosko is known as B3PW91 exchange-correlation functional. The B3PW91 has been employed in other studies of reactivity in copper clusters with good performance,doi:10.1021/acs.jpcc.5b05023 whereas, the PBE exchange-correlation functional PhysRevLett.77.3865 has shown good performace in thermochemistry properties.doi:10.1063/1.3691197 Regarding the LANL2DZ schaefer1977methods basis set, that was used in previous studies of computations of copper-based molecules properties produced result close to experimental values. Legge Not too long ago, in a prevoius DFT studies, the def2-SVP Weigend gave good results in the computations of Cu-metal ligand bond lengths.Niu The true minimun energy structures are validated by the vibrational analysis without imaginary frequency.
II.2 Thermochemistry Properties
All the thermodynamic properties of an ensemble of molecules can be derived from molecular partition functionBuelna ; molecules26133953 ; Dzib ; e20040218 so, the molecular partition function contains all thermodynamic information in a similar way that the quantum wavefunction contains all the information about the system.Buelna ; Dzib Previous theoretical studies used the partition function to compute thermodynamic properties of Cun clusters (n=2, 150) as a function of temperature and demonstrated that the magic clusters is temperature dependentGrigoryan Zhen Hua-Li et al. Truhlar computed the thermodynamics of unsupported neutral Aln () particles evaluating rovibrational partition functions, they reported that the dominant cluster depends on temperature. and gives and overview of recent progress on the nanothermodynamics of metal nanoparticles.Li-Truhlar Christopher Sutton et al. 10.3389/fchem.2020.00757 in framework of atomistic thermodynamics predict the behavior of materials at realistic temperatures. Recently, Buelna-Garcia et al. Buelna ; molecules26133953 used the partition function to compute the temperature-dependent relative population and IR spectra of neutral Be4B8 and anionic Be6B11 clusters, also Dzib et al. Dzib employed a similar procedure to compute the reaction rate constants. Other previous theoretical studies computed the temperature-dependent entropic contibutions on [Fe(pmea)(NCS)2] complex.Brehm In this study, the temperature-dependent Gibbs free energy is computed employing the partition function Q given in Equation, 1 under the rigid rotor, harmonic oscillator, Born-Oppenheimer, ideal gas, and a particle-in-a-box approximations. We have to underline that it must take into account the anharmonicity to compare theory with experiment.D1SC00621E
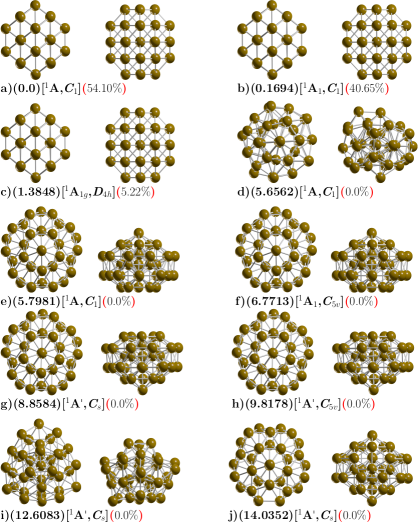
(1) |
In Eq. 1, is the degeneracy factor, is the Boltzmann constant, is the temperature, and is the total energy of a cluster.Buelna ; Dzib ; mcquarrie1975statistical We employ equations 2 to 5 to compute the internal energy (U), enthalpy (H), and Gibbs energy (G) of the Cu38 cluster at finite temperature. The equations to compute entropy contributions (S) are those employed in a previous work10.3389/fchem.2022.841964 ; Buelna ; molecules26133953 ; Dzib and any standard thermodynamics textbook.mcquarrie1975statistical ; hill1986introduction
(2) |
(3) |
(4) |
(5) |
In Equations above, ZPE is the zero-point energy correction is the electronic energy, and are the contributions to energy due to translation, rotation, vibration and electronic as function of temperature, respectively. To compute the Boltzmann probability of occurrence of one particular neutral Cu38 cluster in an ensemble at thermal equilibrium and at finite temperatures, we employ the probability of occurrenceBuelna ; molecules26133953 ; Truhlar ; Bhattacharya ; Bhumla ; Shortle ; MENDOZAWILSON2020112912 ; Dzib ; Schebarchov ; Goldsmith ; Grigoryan ; Truhlar given in Equation 6
(6) |
where , and is the Boltzmann constant, is the temperature, and is the Gibbs free energy of the isomer. We point out that Gibbs free energies must be corrected considering the symmetry, Buelna-Garcia et al. molecules26133953 in a previous work shows that the contribution of the rotational entropy to the Gibbs free energy calculated with and without symmetry behave linearly with the temperature and could be significant 10.3389/fchem.2022.841964 Equation 6 is restricted so that the sum of all probabilities of occurrence, at fixed temperature T, is equal to 1 and given by Equation 7
(7) |
In this study, the Boltzmann weighted UV-Vis spectrum at finite temperature is given by Equation 8
(8) |
Where is the total number of cluster in the ensemble, UV-Visi is the UV-Vis of the isomer at temperature T=0, and Pi(T) is the probability of occurrence of the isomer given by Equation 6. To compute the probability of occurrence and the spectra we used the Boltzmann-Optics-Full-Ader code (BOFA).Buelna ; MENDOZAWILSON2020112912
III Results and Discussion
Isomers (energy kcal/mol) | ||||||||||
---|---|---|---|---|---|---|---|---|---|---|
Level of theory | Energy | |||||||||
0.0 | 0.16 | 1.38 | 5.65 | 5.79 | 5.81 | 6.76 | 8.85 | 9.81 | ||
B3PW91/Def2TZVP/GD3 | 0.0 | 0.09 | 0.0 | 5.01 | 5.01 | 5.01 | 5.01 | 5.01 | 8.17 | |
0.05 | 0.0 | 0.10 | 8.76 | 4.89 | 4.89 | 4.88 | 4.88 | 4.88 | ||
0.0 | 0.95 | 2.0 | 2.40 | 2.73 | 2.91 | 2.94 | 3.28 | 3.32 | ||
B3PW91/Def2TZVP | 0.0 | 0.0 | 2.14 | 3.29 | 6.52 | 3.84 | 2.14 | 3.27 | 3.84 | |
0.0 | 0.0 | 2.09 | 3.27 | 6.13 | 3.76 | 2.09 | 3.28 | 3.76 | ||
0.0 | 0.86 | 0.92 | 5.02 | 7.23 | 7.59 | 7.81 | 8.88 | 12.27 | ||
PBE/Def2TZVP/GD3 | 0.0 | 0.89 | 0.0 | 8.70 | 7.94 | 7.92 | 8.61 | 7.94 | 14.24 | |
0.0 | 0.90 | 0.0 | 9.14 | 7.92 | 7.93 | 8.84 | 7.94 | 12.47 | ||
0.0 | 0.34 | 0.92 | 1.37 | 1.38 | 1.77 | 2.82 | 5.79 | 8.70 | ||
PBE/Def2TZVP | 0.0 | 0.0 | 0.37 | 0.41 | 1.77 | 1.77 | 1.77 | 9.08 | 15.75 | |
0.0 | 0.0 | 0.38 | 0.39 | 1.73 | 1.73 | 1.74 | 9.47 | 9.86 | ||
0.0 | 1.02 | 3.37 | 3.46 | 8.63 | 9.11 | 9.59 | 9.62 | 9.74 | ||
PBE/LANL2DZ/GD3 | 2.03 | 0.0 | 2.12 | 2.14 | 8.31 | 8.77 | 8.73 | 9.34 | 8.71 | |
1.97 | 0.0 | 2.01 | 2.01 | 8.08 | 8.60 | 8.52 | 9.12 | 8.45 | ||
0.0 | 2.15 | 2.87 | 3.03 | 3.26 | 4.31 | 8.89 | 9.66 | 9.85 | ||
PBE/LANL2DZ | 0.97 | 0.0 | 1.46 | 1.38 | 1.64 | 1.68 | 7.98 | 9.19 | 9.58 | |
0.86 | 0.0 | 1.27 | 1.11 | 1.48 | 1.42 | 8.03 | 8.99 | 9.37 |
III.1 The lowest energy structures and energetics
The ball and stick model shown in Figure 1 depicted the lowest-energy structure and the low-energy structures of neutral Cu38 clusters along with some competing isomers. At B3PW91/def2SVP level of theory and taking into account the dispersion pairwise correction of Grimme (DFT-GD3),Grimme ZPE energy corrections and at room temperature and 1 atmospheric pressure. We found a tetrakaidecahedron as the lowest energy structure which has 14 faces: six equivalent square fcc(100) and eight equivalent hexagons, this shape is obtained when cutting the corners off 3D diamond shape, and it is a fcc-like truncated octahedron (TO). The calculated structure belongs to point group symmetry C1, electronic ground state 1A, its lowest IR active vibration frequency is 32.57 cm-1 and is a semiconductor with electronic gap 0.623 eV. It is known that the bulk rare gas crystals have a face centered cubic (FCC) crystalline symmetry.
Previous works on exploration of the potential energy surface of Cu38 cluster using genetic algorithms with Gupta potential often find highly symmetric TO structure,Kostko2005 other reported previous work employing Sutton-Chen potential with monte Carlo simulation also find TO structure.Darby The optimized Cu-Cu bond length is found to be 2.4670, Å which is in good agreement with other reported DFT calculations Cu-Cu dimmerPhysRevA.69.043203 ; PhysRevB.73.155436 of 2.248, Å and is consistent with the experimental bonding distance Cu-Cu 2.22Å. PhysRevA.69.043203 Our computed diameter of TO structure is 7.8 Å and also is in good agreement of 8 Å reported in previous theoretical DFT calculations.PhysRevB.73.155436 The second structure higher in energy lies at 0.16 kcal/mol at temperature of 298.15 K also is a TO structure with point group symmetry C1, electronic ground state 1A, the lowest IR active vibration frequency is 32.13 cm-1, and is a semiconductor with electronic gap 0.623 eV, similarly to that of the putative global minimum. The next structure is slightly higher in energy located at 1.38 kcal/mol also is a TO structure, but with D4h point group symmetry and electronic ground state 1A1g, and the lowest IR active vibration frequency is 33.44 cm-1. We also explored TO structure, initializing the geometry from the high-symmetries OH and TH and after geometry optimization without constrains, the OH and TH symmetries become C1 and DH4 symmetries. The perfect OH symmetry it could be deformed due to the Jahn-Teller effectPhysRevB.73.155436 ; PhysRevA.69.043203 and would have to be taken into account in the calculation of total energyPhysRevB.97.165204 ; ZLATAR201086 and the relative population at temperature T could change as consequence in the optical properties. Opik Recently, in one of our previous works, we clarify the origin of Gibbs free energy differences between two similar structures just with different group symmetry, that is due to the rotational entropy, specifically the factor.molecules26133953 In this work, the energy difference of 0.16 between the two isomers depicted in Figure 1a with symmetry C1 and RMSD, the difference is 0.08 and is due to the Jean teller effect. The structure located at 1.38 kcal/mol above the putative global minimum with D4H symmetry is due to rotational entropy.
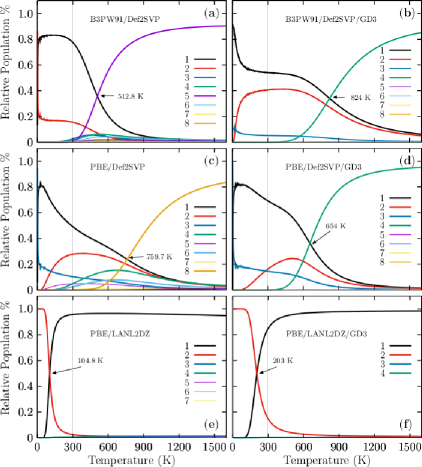
The next structure, shown in Figure 1d is located at 5.65 kcal/mol above the putative global minimum, with point group symmetry C1, and electronic ground state 1A, the lowest IR active vibration frequency is 24.16 cm-1, it is a distorted structure semiconductor with electronic gap of 1.0 eV, the calculated Cu-Cu bond distance is 2.50, Å and the molecular diameter of this structure is 9.1 kcal/mol, slightly larger Cu-Cu bond distance and diameter than the global minimum, this structure possesses the the smallest relative ZPE energy, as shown in Figure, as well as the smallest frequency of the vibrational modes of all isomers.
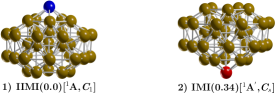
The next two higher energy structures shows in Figure 1(e,f) are located up to 5.8 kcal/mol, an both of them are the incomplete-Mackay icosahedron (IMI) with C1 and C5V point group symmetries and electronic ground state 1A and 1A1 respectively. For both cases, the molecular diameter is 8.54, Å electronic gap of 0.97 eV eV and the Cu-Cu bonding distance is 2.47 Å. There are other higher energy structures shows in Figure 1(g,h,i,j), these do not contribute to any molecular property in all temperature range. In the Supplementary Information, Figure 8, Appendix D, we depicted the lowest energy structures screening at B3PW91/def2SVP level of theory and without taking into account the atom-pairwise correction of Grimme GD3. The lowest energy structure is IMI structure with point group symmetry C1 and electronic ground state 1AH. The molecular diameter is 8.69 Å, slightly larger than those found at TO structure of 7.8, Å the average bond distance is 2.50 Å. At PBE-GD3/Def2SVP level of theory, we found a IMI structure to be the most stable structure whereas at the PBE-GD3/LANL2DZ level of theory we found the TO structure as the putative global minimum energy. The complete description of the structures located at higher energies is in the Supplementary Information. We point out here, for the Cu38 clusters, the order energetically of the isomers and the energy gap among isomers as well as the putative global minimum interchange when we take into account the dispersion interactions. The atomic XYZ coordinates (at B3PW91/def2SVP level of theory) are displayed in Appendix F
III.2 Energetics
In the computation of energies employing different methods yield different results due to the functional and basis sets and therefore the energetic isomer ordering changes.molecules26133953 ; Puente The comparison of two different exchange-correlation functionals and two basis set and with and without taking into acount the dispersion D3 the Grimme are shown in the Table 1. The optimizations performed at the B3PW91/PBE-def2TZVP considering the dispersion yield the same type of lowest energy equilibrium geometry and similar energetic isomer ordering when we employ the electronic energy with and without ZPE correction energy and Gibbs free energy computed at room temperature. From the energetic point of view, the inclusion of dispersion is more important than the type of functional and basis set, i.e The first line of the Table 1 show the relative Gibbs free energies computed at the B3PW91-D3/def2TZVP level of theory, the isomer label ib in the Table 1 and depicted in Figure 1b is located 0.16 kcal/mol above the putative global minimum whereas the second line of the Table 1 show the relative Gibbs free energies computed at the B3PW91/def2TZVP level of theory and the isomer ib in the Table 1 and depicted in Figure 1b is located 0.95 kcal/mol above the putative global minimum as it is shown in Table 1. For isomer ib, the effect of the dispersion on the energy is decrease the relative Gibbs free energy with respect to the putative global minimum. (from 0.95 to 0.16 kcal/mol). The effect of dispersion, in the case of isomers ic, is also decrease the relative Gibbs free energy with respect to the putative global minimum from 2.0 to 1.38 kcal/mol whereas for isomer label id, the relative Gibbs free energy increase from 2.4 to 5.65 kcal/mol. In summary, the effect of dispersion reduces the Gibbs free energy in the lowest energy structures where the Boltzamnn factors are difrentes from zero. A overall comparison of free energies computed with functional B3PW91, second line of the Table 1, and PBE in four line in the Table 1, versus free energies computed with functional PBE, four line of the Table 1, and PBE in four line in the Table 1, shows a reduction in the relative Gibbs free energies when the PBE functional is employed. For the case of the basis set the LANL2DZ increase the relative Gibbs free energy in the low energy isomers as it is shows a comparison of line 6 of Table 1 versus line 1 of Table 1.
III.3 Relative stability
The probability of finding the TO structure with C1 symmetry at B3PW91/def2-SVP level of theory is depicted in black-solid line in panel (a) of Figure 1(4). It strongly dominates from 0 to 300 K, thus all the molecular properties are due only to this structure. From slightly before 300 K, it start to decay exponentially and almost disappear at 900 K, at the same time, the probability of finding the amorphous structure with point group symmetry C1, is depicted in violet-solid line in Figure 2(a), it start to grow exponentially and become dominate at temperature above 512.8 K and at 900 K it become strongly dominate. At solid-solid transition temperature of 512.8 K the TO and the amorphous structure co-exist. The effect of dispersion can be appreciated in panel (b) of Figure 2. The relative population is computed at B3PW91-G3/def2-SVP level of theory. The effect of the dispersion is dramatic, the solid-solid transformation point is shifted from 512.8 to 824 K, an increase of 160% and from the panel (b) one can see that the molecular properties below 600 K, are due to only the TO structure. Slightly before 600 K, The probability of finding the amorphous structure, depicted in green-solid line in panel (b), start to grow up exponentially and at temperature of 824 K the TO and amorphous structure co-exit. Whereas a temperature of 600 K the probability of find the TO structure start do decay exponentially and at 900 K its value still around 20%. The probabilities of occurrence at the PBE/def2-SVP level of theory of a particular Cu38 isomer are displayed in panel (c) of Figure 2. The dominant putative global minimum structure at T=0, is the inverted incomplete-Mackay icosahedron (IIMI) structure depicted in Figure 3a with C1 symmetry and its probrobability of finding it is depicted as a black solid line in panel (c) of Figure 2. Its probability decays almost linearly until 1000 K where it almost disappears. At temperature of 759.7 K, the solid-solid transformation point, the IIMI structure co-exist with an amorphous structure. The probability of finding the amorphous structure start to grew up at 600 K, and above of solid-solid transformation point, it start to strongly dominate as putative global minimum. Where the probability of finding the IMI structure is depicted in red-solid line on panel (c) of Figure 2, the largest probability is 30% at room temperature. Interesting, the probabilities of IIMI and IMI structures does not cross at cold temperature. Zhang et al. Zhang2019 reported that IMI structure could be highly competitive at finite temperatures, but our findings shows that the amorphous structure with C1 symmetry is highly dominant at hot temperatures, whereas the IIMI structure is highly dominant at cold temperatures.
For ease comparison, the Figure 3 display the IIMI and the IMI structures side by side. At cold and the IIMI structure dominate. The effects of dispersion is shift the solid-solid transformation point to lower temperatures from 759.7 K to 654 K as one can see in panel (d) Figure 2. The probability of finding the IIMI structure as a function of temperature is depicted in black solid-line and it decays approximately linearly from 50 to 500 K, after that it decays exponentially until 900 K where it disappears. At around 400 K, the probability of finding the amorphous structure, depicted in green solid-line in panel (d) of Figure 2, start to grow up in exponential way, and at temperature of 654 K it co-exit with the IIMI structure. Above 654 K, the amorphous structure becomes energetically favorable.
III.4 IR spectra at finite temperature
The properties observed in a molecule are statistical averages over the ensemble of geometrical conformations or isomers accessible to the cluster, so the molecular properties are governed by the Boltzmann distributions of isomers that can change significantly with the temperature primary due to entropic effects.molecules26133953 ; Buelna ; Truhlar The major contributions to the entropy are the many soft vibrational modes that the clusters possesses. The IR spectrum is related to vibrations or rotations that alter the dipole moment, and it will happen in molecules that have a dipole moment. Also, the IR spectrum is related to the curvature of the potential curve versus interatomic distances. Complete information about molecular vibrations allows us to analyze catalytic chemical reactions.TINNEMANS20063 ; BRANDHORST200634 ; Hashimoto2019 IR spectra are used to identify functional groups and chemical bond information. However, assigning IR bands to vibrational molecular modes in the measured spectra can be difficult and requires DFT calculations; as we mentioned earlier, the temperature is not considered in these computations and discrepancies between experimental and computed IR spectra can result from finite temperature, anharmonic effects, and multi-photon nature of experments, whereas IR computations assume single-photon processes.Buelna ; molecules26133953 The IR spectra of isolated metal clusters in the gas phase for vanadium cluster cations as well as for neutral and cationic niobium clusters were measured.Fielicke2005 Even though Cu clusters are important in catalysis and were the first clusters experimentally produced,doi:10.1021/j100211a002 the available structural information is limited to study photoelectron spectroscopy for anions, mass spectrometry, and photodissociation spectra in the visible range.doi:10.1021/acs.jpclett.9b00539 Reciently, Lushchikova et al. doi:10.1021/acs.jpclett.9b00539 determine the structure of small cationic copper clusters based on a combination of IR spectroscopy of Cu-Arm complexes and DFT calculations. In this work, the IR spectra of isomers computations were carried out using the Gaussian package under harmonic approximation at level of theory PBPW91-D3YANAI200451 /def2TZVP and full width at half maximum (FWHM) of 8 cm-1 taking into account the dispersion of Grimme D3 as implemented in Gaussian code. Negative frequencies were checked in all calculations to ensure that there were not transitions states. The computed frequencies were scaled by 0.98 to estimate the observed frequencies. Here, the total IR spectrum is computed as a weighted Boltzmann sum of the single IR spectrum of each isomer of the distribution at finite temperaturemolecules26133953 ; Buelna ; doi:10.1063/1.3552077 ; PhysRevA.70.041201 given by Equation 8 and the probabilities of occurrence displayed in Figure 2. To our knowledge, there are a few theoretical studies on IR spectra of noble metal clusters computed considering a weighted sum of the IR spectra of isomers. PhysRevA.70.041201 Computed weighted Boltzmann IR spectra of Cu38 clusters at different temperatures are shown in Figure 6, Appendix B. Notice that the transition metal clusters are very stable, and its vibrational frequencies are found below 400 cm-1doi:10.1063/1.4822324 in good agreement with our computed spectra displayed in Figure 6, Appendix B.
III.5 UV-Visible spectra at finite temperature
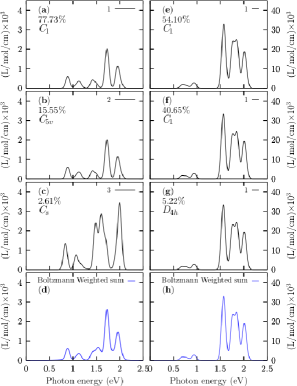
The optical properties are a source of atomic structural information, and their electronic structure determines them.ma13194300 In this paper the TD-DFT was used to compute the optical absorption spectra in the UV-visible range eV for the Cu38 cluster. We employ the CAM-B3LYP functional, Def2-TZVP basis set, and 50 singlets and 50 triplets states. The transition metals clusters allow us to study the influence of the d electrons on the optical properties; it is known that the d electrons strongly influence the surface plasmon response.doi:10.1063/1.3552077 We show in Figure 4 the UV-spectra for the Cu38 cluster with and without the Grimme’s dispersion D3. To take into account the effects of temperature on the UV-visible spectra. We consider that the UV-visible spectrum of a molecular ensemble is a weighted sum of all the individual contributions of each isomer that forms the ensemble. In panel labeled (e) is displayed the UV spectra considering the Grimme’s dispersion GD3 and for the lowest energy structure with symmetry C1; this structure contribute with 54% to the total Boltzamnn spectrum. In panel (f) is displayed the UV-visible for the low-energy structure located at 0.16 kcal/mol at room temperature and above the putative global minimum with C1 symmetry, and this contributes to 40% to the Boltzmann spectrum. In panel (g) is displayed the UV-visible for the low-energy structure located at 1.38 kcal/mol at room temperature and above the putative global minimum with D4h symmetry, and this contributes to 5.22% to the Boltzmann spectrum. In panel (h) is displayed the Boltzmann weighted UV-Visible spectrum at room temperature. Notice that all absorption spectra, (g) to (h), are similar; The Boltzmann weighted UV-Visible spectrum presented in Figure 4, panel (h) is composed of three peaks located between 1.5 to 2 eV and smaller intensity peaks located at 0.5 and 1.0 eV. The most significant absorption peak is located at 1.6 eV. Our computations show five absorption peaks of the UV-visible spectrum starting at 0.5 eV and finishing at 2.5 eV. Notice that the total optical spectrum is due only to the putative global minimum; despite the number of isomers growing exponentially, the main contribution to the optical properties comes from those low energy structures very close to the global minimum where weights Boltzmann factors temperature dependents are different from zero. Interestingly, the second low-energy structure located at 0.16 kcal/mol (room temperature) above the putative lowest energy structure screen or blocked the contributions to the Boltzmann optical spectrum of the other structures. In this paper, we called those structures as shielding structures. In Figure 4, panels (a) to (d) are displayed the optical absorption spectra in the UV-visible range computed with structures optimized without the Grimme’s dispersion D3. Notice the effects of dispersion on UV-visible spectra is introduced by the change of the relative population when the optimizations are computed without, and with dispersion, e.g., the contribution, to the Boltzmann weighted UV-Visible spectrum, of the lowest energy structure computed without Grimme’s dispersion D3 is 77% meanwhile, the contribution is 54% when an optimized structure is computed with Grimme’s dispersion D3. The complete evolution of the Boltzmann weighted UV-Visible spectra for temperatures ranging from 10 to 1500 K is displayed in Figure 7, Appendix C.
III.6 Molecular Dynamics
We performed Born-Oppenheimer molecular dynamics (MD) of the Cu38 cluster employing the deMon2K programdemon2k (deMon2k v. 6.01, Cinvestav, Mexico City, Mexico, 2011) at temperature of 600 K, aiming to gain insight into the dynamical behavior of the Cu38 cluster. The MD started from global putative minimun structure. The simulation time was 25 ps with a step size of 1 fs. All computations were performed under scheme of ADFT,doi:10.1063/1.1771638 and for the basis set we employed the double zeta plus valence polarization (DZVP) all-electron basis. We employed the Nosé-Hoover thermostat to fix the temperature and the linear and angular momenta of the cluster Cu38 were initialized to zero and conserved.
IV Conclusions
For the first time, to our knowledge and from our results, we proposed the inverted incomplete-Mackay icosahedron (IIMI) with symmetry C1 as low-energy structure than the incomplete-Mackay icosahedron (IMI) with symmetry Cs and located 0.34 kcal/mol energy above the putative minimum global at 298.15 K. The yellow, the IIMI structure is the result of interchanging the red Cu atom depicted in the IMI structure to the position of blue atom in the IIMI structure. The IMI structure was reported in referenceZhang2019 as the low-energy structure. The HOMO-LUMO gap of the IMI structure is 0.24 eV (0.356 eV reported by previous DFT studiesZhang2019 ) whereas for the HOMO-LUMO gap for IIMI structure is 0.30 eV, suggesting why the IIMI structure is energetically more stable. We computed the effect of symmetry on the Boltzmann populations on Cu38 clusters. In spite that the number of isomers grows exponentially, the main contribution to the optical properties comes from those low energy structures very close to the global minimum where weights Boltzmann factors temperature dependents are different from zero. We performed an unbiased global search for minimum energy Cu38 clusters structures using a two-stage strategy. First a global search using a semi empirical methodology, followed by a density functional theory optimization of the best structures from the first stage was done at different levels of theory. The temperature and entropic effects cause several competing structures because energy separation between isomers on the free energy surface is small and changes the dominant structure, so probably a mixture of isomers interconverted at temperature finite. Those energetically competing structures provide a different percentage of the entire IR spectrum. On the other hand, those higher energy structures with significant energy separation among isomers on the potential energy surface do not contribute to the entire IR spectrum. Despite that, the number of isomers grows exponentially. The main contribution to the molecular properties comes from those low-energy structures very close to the global minimum, where weight’s Boltzmann factors temperature dependents are different from zero. (depends strongly on the energy separation, if the energy separation is significant, the IR spectrum going to be rigid, not changes) A motif is dominant in cold conditions, whereas the other motif is dominant in other hot conditions; additionally, the level of theory is decisive in the computations of TSS point dependent-temperature Our computations clearly show (relative population) that the low-symmetry isomers become more stable at high temperatures due to the entropic effect and the fact that energy states of molecules follow Boltzmann distribution in all six different levels of theory. Our unbiased global search on the free energy surface show that there is an amorphous structure that strongly dominate at hot temperatures, as far as we know this is a novel putative global minimum at hot temperatures. As immediate work is the computations of the relative populations at high level of theory and compute the diagnostic to determine that the computed DFT energies are not properly described by a single reference method or contain a multireference character. So, further studies needed to be done.
V Acknowledgments
C. E. B.-G. thanks Conacyt for the Ph.D. scholarship (860052). E. P.-S. thanks Conacyt for the Ph.D. scholarship (1008864). We are grateful Universidad Politécnica de Tapachula (UPTap) for granting us access to their clusters and computational support. We are also grateful to the computational chemistry laboratory and ICEME research group at UPtap for providing computational resources, ELBAKYAN, and PAKAL supercomputers.
VI Conflicts of Interest
The authors declare no conflict of interest.
VII Funding
This research received no external funding.
VIII Abbreviations
The following abbreviations are used in this manuscript:
Density Functional Theory (DFT)
Zero-Point Energy (ZPE)
References
- [1] J. P. Wilcoxon and B. L. Abrams. Synthesis, structure and properties of metal nanoclusters. Chem. Soc. Rev., 35:1162–1194, 2006.
- [2] Riccardo Ferrando, Julius Jellinek, and Roy L. Johnston. Nanoalloys: From theory to applications of alloy clusters and nanoparticles. Chemical Reviews, 108(3):845–910, 2008.
- [3] Gajendra Kumar Inwati, Yashvant Rao, and Man Singh. Thermodynamically induced in situ and tunable cu plasmonic behaviour. Scientific Reports, 8(1):3006, Feb 2018.
- [4] Ammu Mathew and Thalappil Pradeep. Noble metal clusters: Applications in energy, environment, and biology. Particle & Particle Systems Characterization, 31(10):1017–1053, 2014.
- [5] Paulrajpillai Lourdu Xavier, Kamalesh Chaudhari, Ananya Baksi, and Thalappil Pradeep. Protein-protected luminescent noble metal quantum clusters: an emerging trend in atomic cluster nanoscience. Nano reviews, 3:10.3402/nano.v3i0.14767, 2012.
- [6] Chuanchuan Zhang, Haiming Duan, Xin Lv, Biaobing Cao, Ablat Abliz, Zhaofeng Wu, and Mengqiu Long. Static and dynamical isomerization of Cu38 cluster. Scientific Reports, 9(1):7564, May 2019.
- [7] Peisheng Liu, Hao Wang, Xiaoming Li, Muchen Rui, and Haibo Zeng. Localized surface plasmon resonance of Cu nanoparticles by laser ablation in liquid media. RSC Adv., 5:79738–79745, 2015.
- [8] Puru Jena and A. W. Castleman. Clusters: A bridge across the disciplines of physics and chemistry. Proceedings of the National Academy of Sciences, 103(28):10560–10569, 2006.
- [9] Dung T. Tran and Roy L. Johnston. Theoretical study of CuAun clusters using a combined empirical potential–density functional approach. Phys. Chem. Chem. Phys., 11:10340–10349, 2009.
- [10] Francesca Baletto, Arnaldo Rapallo, Giulia Rossi, and Riccardo Ferrando. Dynamical effects in the formation of magic cluster structures. Phys. Rev. B, 69:235421, Jun 2004.
- [11] Walt A. de Heer. The physics of simple metal clusters: experimental aspects and simple models. Rev. Mod. Phys., 65:611–676, Jul 1993.
- [12] Jonathan P. K. Doye and David J. Wales. Global minima for transition metal clusters described by sutton-chen potentials. New J. Chem., 22:733–744, 1998.
- [13] C. L. Pettiette, S. H. Yang, M. J. Craycraft, J. Conceicao, R. T. Laaksonen, O. Cheshnovsky, and R. E. Smalley. Ultraviolet photoelectron spectroscopy of copper clusters. The Journal of Chemical Physics, 88(9):5377–5382, 1988.
- [14] O. Kostko, N. Morgner, M. Astruc Hoffmann, and B. von Issendorff. Photoelectron spectra of Nan- and Cun- with n = 20–40: observation ofsurprising similarities. The European Physical Journal D - Atomic, Molecular, Optical and Plasma Physics, 34(1):133–137, Jul 2005.
- [15] Nobuhisa Fujima and Tsuyoshi Yamaguchi. Magnetic anomaly and shell structure of electronic states of nickel microclusters. Journal of the Physical Society of Japan, 58(9):3290–3297, 1989.
- [16] Christopher D. Taylor, Matthew Neurock, and John R. Scully. First-principles investigation of the fundamental corrosion properties of a model Cu38 nanoparticle and the (111), (113) surfaces. Journal of The Electrochemical Society, 155(8):C407, 2008.
- [17] Dabin Qi, Xudong Luo, Jun Yao, Xiaojun Lu, and Zhan Zhang. Computational study of reverse water gas shift reaction on Cu38 cluster model and cu slab model. Journal of Theoretical and Computational Chemistry, 19(02):2050008, 2020.
- [18] Nozomi Takagi, Kazuya Ishimura, Masafuyu Matsui, Ryoichi Fukuda, Masahiro Ehara, and Shigeyoshi Sakaki. Core-shell versus other structures in binary Cu38-nMn nanoclusters (M = Ru, Rh, Pd, Ag, Os, Ir, Pt, and Au; n = 1, 2, and 6): Theoretical insight into determining factors. The Journal of Physical Chemistry C, 121(19):10514–10528, 2017.
- [19] John P. Perdew, J. A. Chevary, S. H. Vosko, Koblar A. Jackson, Mark R. Pederson, D. J. Singh, and Carlos Fiolhais. Atoms, molecules, solids, and surfaces: Applications of the generalized gradient approximation for exchange and correlation. Phys. Rev. B, 46:6671–6687, Sep 1992.
- [20] Masahiro Itoh, Vijay Kumar, Tadafumi Adschiri, and Yoshiyuki Kawazoe. Comprehensive study of sodium, copper, and silver clusters over a wide range of sizes 2n75. The Journal of Chemical Physics, 131(17):174510, 2009.
- [21] I. A. Hijazi and Y. H. Park. Structure of pure metallic nanoclusters: Monte carlo simulation and ab initio study. The European Physical Journal D, 59(2):215–221, Aug 2010.
- [22] V. G. Grigoryan, D. Alamanova, and M. Springborg. Structure and energetics of nickel, copper, and gold clusters. The European Physical Journal D - Atomic, Molecular, Optical and Plasma Physics, 34(1):187–190, Jul 2005.
- [23] Şakir Erkoç and Riad Shaltaf. Monte carlo computer simulation of copper clusters. Phys. Rev. A, 60:3053–3057, Oct 1999.
- [24] ŞSakir Erkoç. An empirical many-body potential energy function constructed from pair-interactions. Zeitschrift für Physik D Atoms, Molecules and Clusters, 32(3):257–260, Sep 1994.
- [25] Valeri G. Grigoryan, Denitsa Alamanova, and Michael Springborg. Structure and energetics of clusters with : An embedded-atom-method study. Phys. Rev. B, 73:115415, Mar 2006.
- [26] Bo Zhao, Riguang Zhang, Zaixing Huang, and Baojun Wang. Effect of the size of Cu clusters on selectivity and activity of acetylene selective hydrogenation. Applied Catalysis A: General, 546:111–121, 2017.
- [27] Estefanía Fernández, Mercedes Boronat, and Avelino Corma. Trends in the reactivity of molecular O2 with copper clusters: Influence of size and shape. The Journal of Physical Chemistry C, 119(34):19832–19846, 2015.
- [28] Sarah Darby, Thomas V. Mortimer-Jones, Roy L. Johnston, and Christopher Roberts. Theoretical study of Cu-Au nanoalloy clusters using a genetic algorithm. The Journal of Chemical Physics, 116(4):1536–1550, 2002.
- [29] S. Núnez and R. L. Johnston. Structures and chemical ordering of small Cu-Ag clusters. The Journal of Physical Chemistry C, 114(31):13255–13266, 2010.
- [30] Young Ho Park and Iyad A. Hijazi. Critical size of transitional copper clusters for ground state structure determination: empirical and ab initio study. Molecular Simulation, 38(3):241–247, 2012.
- [31] Carlos Emiliano Buelna-Garcia, José Luis Cabellos, Jesus Manuel Quiroz-Castillo, Gerardo Martinez-Guajardo, Cesar Castillo-Quevedo, Aned de Leon-Flores, Gilberto Anzueto-Sanchez, and Martha Fabiola Martin-del Campo-Solis. Exploration of free energy surface and thermal effects on relative population and infrared spectrum of the Be6B fluxional cluster. Materials, 14(1), 2021.
- [32] Cesar Castillo-Quevedo, Carlos Emiliano Buelna-Garcia, Edgar Paredes-Sotelo, Eduardo Robles-Chaparro, Edgar Zamora-Gonzalez, Martha Fabiola Martin-del Campo-Solis, Jesus Manuel Quiroz-Castillo, Teresa del Castillo-Castro, Gerardo Martínez-Guajardo, Aned de Leon-Flores, Manuel Cortez-Valadez, Filiberto Ortiz-Chi, Tulio Gaxiola, Santos Jesus Castillo, Alejandro Vásquez-Espinal, Sudip Pan, and Jose Luis Cabellos. Effects of temperature on enantiomerization energy and distribution of isomers in the chiral Cu13 cluster. Molecules, 26(18), 2021.
- [33] E. de la Puente, A. Aguado, A. Ayuela, and J. M. López. Structural and electronic properties of small neutral clusters. Phys. Rev. B, 56:7607–7614, Sep 1997.
- [34] Nicola Marzari, David Vanderbilt, and M. C. Payne. Ensemble density-functional theory for ab initio molecular dynamics of metals and finite-temperature insulators. Phys. Rev. Lett., 79:1337–1340, Aug 1997.
- [35] Ana María Mendoza-Wilson, René Renato Balandrán-Quintana, and José Luis Cabellos. Thermochemical behavior of sorghum procyanidin trimers with C4-C8 and C4-C6 interflavan bonds in the reaction with superoxide anion radical and H2O2-forming NADH-oxidase flavoenzyme. Computational and Theoretical Chemistry, 1186:112912, 2020.
- [36] Carlos Emiliano Buelna-García, Eduardo Robles-Chaparro, Tristan Parra-Arellano, Jesus Manuel Quiroz-Castillo, Teresa del Castillo-Castro, Gerardo Martínez-Guajardo, Cesar Castillo-Quevedo, Aned de León-Flores, Gilberto Anzueto-Sánchez, Martha Fabiola Martin-del Campo-Solis, Ana Maria Mendoza-Wilson, Alejandro Vásquez-Espinal, and Jose Luis Cabellos. Theoretical prediction of structures, vibrational circular dichroism, and infrared spectra of chiral Be4B8 cluster at different temperatures. Molecules, 26(13), 2021.
- [37] L. V. Redel, Yu. Ya. Gafner, and S. L. Gafner. Role of magic numbers in structure formation in small silver nanoclusters. Physics of the Solid State, 57(10):2117–2125, Oct 2015.
- [38] Terrell L. Hill. Thermodynamics of small systems. The Journal of Chemical Physics, 36(12):3182–3197, 1962.
- [39] Zhen Hua Li and Donald G. Truhlar. Nanothermodynamics of metal nanoparticles. Chem. Sci., 5:2605–2624, 2014.
- [40] Zhen Hua Li, Ahren W. Jasper, and Donald G. Truhlar. Structures, rugged energetic landscapes, and nanothermodynamics of Aln (2n65) particles. Journal of the American Chemical Society, 129(48):14899–14910, 2007. PMID: 17994736.
- [41] Francesca Baletto and Riccardo Ferrando. Structural properties of nanoclusters: Energetic, thermodynamic, and kinetic effects. Rev. Mod. Phys., 77:371–423, May 2005.
- [42] Valeri G. Grigoryan and Michael Springborg. Temperature and isomeric effects in nanoclusters. Phys. Chem. Chem. Phys., 21:5646–5654, 2019.
- [43] M. Bixon and Joshua Jortner. Energetic and thermodynamic size effects in molecular clusters. The Journal of Chemical Physics, 91(3):1631–1642, 1989.
- [44] Florent Calvo. Thermodynamics of nanoalloys. Phys. Chem. Chem. Phys., 17:27922–27939, 2015.
- [45] David J. Wales. Structure, dynamics, and thermodynamics of clusters: Tales from topographic potential surfaces. Science, 271(5251):925–929, 1996.
- [46] Gerardo Martínez-Guajardo, Jose Luis Cabellos, Andres Díaz-Celaya, Sudip Pan, Rafael Islas, Pratim K. Chattaraj, Thomas Heine, and Gabriel Merino. Dynamical behavior of borospherene: A nanobubble. Sci. Report, 22:11287–11297, June 2015.
- [47] Koichi Ohno and Satoshi Maeda. Global reaction route mapping on potential energy surfaces of formaldehyde, formic acid, and their metal-substituted analogues. The Journal of Physical Chemistry A, 110(28):8933–8941, 2006. PMID: 16836457.
- [48] Carlos Emilano Buelna-Garcia, Cesar Castillo-Quevedo, Edgar Paredes-Sotelo, Gerardo Martinez-Guajardo, and Jose Luis Cabellos. Boltzmann populations of the fluxional Be6B and chiral Be4B8 clusters at finite temperatures computed by DFT and statistical thermodynamics. IntechOpen: Doi:10.5772/intechopen.100771, 2021.
- [49] Uzi Even, Narda Ben-Horin, and Joshua Jortner. Multistate isomerization of size-selected clusters. Phys. Rev. Lett., 62:140–143, Jan 1989.
- [50] Carlos Emiliano Buelna-García, Cesar Castillo-Quevedo, Jesus Manuel Quiroz-Castillo, Edgar Paredes-Sotelo, Manuel Cortez-Valadez, Martha Fabiola Martin-del Campo-Solis, Tzarara López-Luke, Marycarmen Utrilla-Vázquez, Ana Maria Mendoza-Wilson, Peter L. Rodríguez-Kessler, Alejandro Vazquez-Espinal, Sudip Pan, Aned de Leon-Flores, Jhonny Robert Mis-May, Adán R. Rodríguez-Domínguez, Gerardo Martínez-Guajardo, and Jose Luis Cabellos. Relative populations and IR spectra of Cu38 cluster at finite temperature based on DFT and statistical thermodynamics calculations. Frontiers in Chemistry, 10, 2022.
- [51] Christopher Sutton and Sergey V. Levchenko. First-principles atomistic thermodynamics and configurational entropy. Frontiers in Chemistry, 8:757, 2020.
- [52] Peter Kratzer and Jorg Neugebauer. The basics of electronic structure theory for periodic systems. Frontiers in Chemistry, 7, 2019.
- [53] Chris J Pickard and R J Needs. Ab initio random structure searching. Journal of Physics: Condensed Matter, 23(5):053201, jan 2011.
- [54] S. Kirkpatrick, C. D. Gelatt, and M. P. Vecchi. Optimization by simulated annealing. Science, 220(4598):671–680, 1983.
- [55] Nicholas Metropolis, Arianna W. Rosenbluth, Marshall N. Rosenbluth, Augusta H. Teller, and Edward Teller. Equation of state calculations by fast computing machines. J. Chem. Phys., 21(6):1087–1092, 1953.
- [56] Y. Xiang and X. G. Gong. Efficiency of generalized simulated annealing. Phys. Rev. E, 62:4473–4476, Sep 2000.
- [57] Yang Xiang, Sylvain Gubian, Brian Suomela, and Julia Hoeng. Generalized simulated annealing for global optimization: the GenSA package for R. The R Journal, 5(1):13–29, June 2013.
- [58] D.G. Vlachos, L.D. Schmidt, and R. Aris. Comparison of small metal clusters: Ni, Pd, Pt, Cu, Ag, Au. Z. Phys. D Atom Mol. Cl., 26(1):156–158, 1993.
- [59] V. Granville, M. Krivanek, and J.-P. Rasson. Simulated annealing: a proof of convergence. IEEE Trans. Pattern Anal. Mach. Intell., 16(6):652–656, 1994.
- [60] Sudip Pan, Diego Moreno, Jose Luis Cabellos, Jonathan Romero, Andres Reyes, Gabriel Merino, and Pratim K. Chattaraj. In quest of strong Be-Ng bonds among the neutral Ng-Be complexes. The Journal of Physical Chemistry A, 118(2):487–494, 2014. PMID: 24199587.
- [61] Zhong-hua Cui, Yi-hong Ding, Jose Luis Cabellos, Edison Osorio, Rafael Islas, Albeiro Restrepo, and Gabriel Merino. Planar tetracoordinate carbons with a double bond in CAl3E clusters. Phys. Chem. Chem. Phys., 17:8769–8775, 2015.
- [62] Alba Vargas-Caamal, Sudip Pan, Filiberto Ortiz-Chi, Jose Luis Cabellos, Roberto A. Boto, Julia Contreras-Garcia, Albeiro Restrepo, Pratim K. Chattaraj, and Gabriel Merino. How strong are the metallocene–metallocene interactions? cases of ferrocene, ruthenocene, and osmocene. Phys. Chem. Chem. Phys., 18:550–556, 2016.
- [63] Alba Vargas-Caamal, Jose Luis Cabellos, Filiberto Ortiz-Chi, Henry S. Rzepa, Albeiro Restrepo, and Gabriel Merino. How many water molecules does it take to dissociate HCl? Chemistry – A European Journal, 22(8):2812–2818, 2016.
- [64] Zhong-hua Cui, Valentin Vassilev-Galindo, Jose Luis Cabellos, Edison Osorio, Mesías Orozco, Sudip Pan, Yi-hong Ding, and Gabriel Merino. Planar pentacoordinate carbon atoms embedded in a metallocene framework. Chem. Commun., 53:138–141, 2017.
- [65] Alba Vargas-Caamal, Filiberto Ortiz-Chi, Diego Moreno, Albeiro Restrepo, Gabriel Merino, and Jose Luis Cabellos. The rich and complex potential energy surface of the ethanol dimer. Theoretical Chemistry Accounts, 134(2):16, 2015.
- [66] Elizabeth Flórez, Nancy Acelas, César Ibargüen, Sukanta Mondal, Jose Luis Cabellos, Gabriel Merino, and Albeiro Restrepo. Microsolvation of NO: structural exploration and bonding analysis. RSC Adv., 6:71913–71923, 2016.
- [67] Estefanía Ravell, Said Jalife, Jorge Barroso, Mesias Orozco-Ic, Gerardo Hernandez-Juarez, Filiberto Ortiz-Chi, Sudip Pan, Jose Luis Cabellos, and Gabriel Merino. Structure and bonding in CE5- (E=Al-Tl) clusters: Planar tetracoordinate carbon versus pentacoordinate carbon. Chemistry - An Asian Journal, 13(11):1467–1473, 2018.
- [68] C. Z. Hadad, Elizabeth Florez, Gabriel Merino, José Luis Cabellos, Franklin Ferraro, and Albeiro Restrepo. Potential energy surfaces of WC6 clusters in different spin states. The Journal of Physical Chemistry A, 118(31):5762–5768, 2014. PMID: 24533862.
- [69] Martin Saunders. Stochastic search for isomers on a quantum mechanical surface. Journal of Computational Chemistry, 25(5):621–626, 2004.
- [70] Martin Saunders. Stochastic exploration of molecular mechanics energy surfaces. hunting for the global minimum. Journal of the American Chemical Society, 109(10):3150–3152, 1987.
- [71] Rafael Grande-Aztatzi, Paulina R. Martínez-Alanis, José Luis Cabellos, Edison Osorio, Ana Martínez, and Gabriel Merino. Structural evolution of small gold clusters doped by one and two boron atoms. Journal of Computational Chemistry, 35(32):2288–2296, 2014.
- [72] Jin-Chang Guo, Lin-Yan Feng, Ying-Jin Wang, Said Jalife, Alejandro Vásquez-Espinal, Jose Luis Cabellos, Sudip Pan, Gabriel Merino, and Hua-Jin Zhai. Coaxial triple-layered versus helical Be6B clusters: Dual structural fluxionality and multifold aromaticity. Angewandte Chemie International Edition, 56(34):10174–10177, 2017.
- [73] Xue Dong, Said Jalife, Alejandro Vásquez-Espinal, Estefanía Ravell, Sudip Pan, José Luis Cabellos, Wei-yan Liang, Zhong-hua Cui, and Gabriel Merino. Li2B12 and Li3B12: Prediction of the smallest tubular and Cage-like boron structures. Angewandte Chemie International Edition, 57(17):4627–4631, 2018.
- [74] Sukanta Mondal, Jose Luis Cabellos, Sudip Pan, Edison Osorio, Juan Jose Torres-Vega, William Tiznado, Albeiro Restrepo, and Gabriel Merino. 10--electron arenes a la carte: structure and bonding of the [E-(CnHn)-E]n-6 (E = Ca, Sr, Ba; n = 6-8) complexes. Phys. Chem. Chem. Phys., 18:11909–11918, 2016.
- [75] P. L. Rodríguez-Kessler, Sudip Pan, Elizabeth Florez, José Luis Cabellos, and Gabriel Merino. Structural evolution of the rhodium-doped silver clusters AgnRh (n15) and their reactivity toward no. The Journal of Physical Chemistry C, 121(35):19420–19427, 2017.
- [76] Anastassia N. Alexandrova, Alexander I. Boldyrev, You-Jun Fu, Xin Yang, Xue-Bin Wang, and Lai-Sheng Wang. Structure of the NaxClx (x=1,4) clusters via ab-initio genetic algorithm and photoelectron spectroscopy. J. Chem. Phys., 121(12):5709–5719, 2004.
- [77] Nicholas T. Wilson and Roy L. Johnston. A theoretical study of atom ordering in copper–gold nanoalloy clusters. J. Mater. Chem., 12:2913–2922, 2002.
- [78] Fabrizio Cleri and Vittorio Rosato. Tight-binding potentials for transition metals and alloys. Phys. Rev. B, 48:22–33, Jul 1993.
- [79] M. J. Frisch, G. W. Trucks, H. B. Schlegel, G. E. Scuseria, M. A. Robb, J. R. Cheeseman, G. Scalmani, V. Barone, B. Mennucci, G. A. Petersson, H. Nakatsuji, M. Caricato, X. Li, H. P. Hratchian, A. F. Izmaylov, J. Bloino, G. Zheng, J. L. Sonnenberg, M. Hada, M. Ehara, K. Toyota, R. Fukuda, J. Hasegawa, M. Ishida, T. Nakajima, Y. Honda, O. Kitao, H. Nakai, T. Vreven, J. A. Montgomery, J. E. Peralta, F. Ogliaro, M. Bearpark, J. J. Heyd, E. Brothers, K. N. Kudin, V. N. Staroverov, R. Kobayashi, J. Normand, K. Raghavachari, A. Rendell, J. C. Burant, S. S. Iyengar, J. Tomasi, M. Cossi, N. Rega, J. M. Millam, M. Klene, J. E. Knox, J. B. Cross, V. Bakken, C. Adamo, J. Jaramillo, R. Gomperts, R. E. Stratmann, O. Yazyev, A. J. Austin, R. Cammi, C. Pomelli, J. W. Ochterski, R. L. Martin, K. Morokuma, V. G. Zakrzewski, G. A. Voth, P. Salvador, J. J. Dannenberg, S. Dapprich, A. D. Daniels, Farkas, J. B. Foresman, J. V. Ortiz, J. Cioslowski, and D. J. Fox. Gaussian 09, 2009.
- [80] Stefan Grimme, Jens Antony, Stephan Ehrlich, and Helge Krieg. A consistent and accurate ab initio parametrization of density functional dispersion correction (DFT-D) for the 94 elements H-Pu. The Journal of Chemical Physics, 132(15):154104, 2010.
- [81] Axel D. Becke. Density‐functional thermochemistry III. the role of exact exchange. The Journal of Chemical Physics, 98(7):5648–5652, 1993.
- [82] A. D. Becke. Density-functional exchange-energy approximation with correct asymptotic behavior. Phys. Rev. A, 38:3098–3100, Sep 1988.
- [83] John P. Perdew and Yue Wang. Accurate and simple analytic representation of the electron-gas correlation energy. Phys. Rev. B, 45:13244–13249, Jun 1992.
- [84] John P. Perdew, Kieron Burke, and Matthias Ernzerhof. Generalized gradient approximation made simple. Phys. Rev. Lett., 77:3865–3868, Oct 1996.
- [85] Jorge M. del Campo, José L. Gázquez, S. B. Trickey, and Alberto Vela. Non-empirical improvement of PBE and its hybrid PBE0 for general description of molecular properties. The Journal of Chemical Physics, 136(10):104108, 2012.
- [86] T. H. Jr. Dunning and P. J. Hay. Modern theoretical chemistry vol. 3. In H. F Schaefer-III, editor, Methods of Electronic Structure Theory, chapter 1, pages 1–28. Springer US, Plenum, New York, 1977.
- [87] F. Sue Legge, Graeme L. Nyberg, and J. Barrie Peel. DFT calculations for Cu-, Ag-, and Au-containing molecules. The Journal of Physical Chemistry A, 105(33):7905–7916, 2001.
- [88] Florian Weigend and Reinhart Ahlrichs. Balanced basis sets of split valence, triple zeta valence and quadruple zeta valence quality for H to Rn: Design and assessment of accuracy. Phys. Chem. Chem. Phys., 7:3297–3305, 2005.
- [89] Shuqiang Niu, Dao-Ling Huang, Phuong D. Dau, Hong-Tao Liu, Lai-Sheng Wang, and Toshiko Ichiye. Assessment of quantum mechanical methods for copper and iron complexes by photoelectron spectroscopy. Journal of Chemical Theory and Computation, 10(3):1283–1291, 2014. PMID: 24803858.
- [90] Eugenia Dzib, José Luis Cabellos, Filiberto Ortíz-Chi, Sudip Pan, Annia Galano, and Gabriel Merino. Eyringpy: A program for computing rate constants in the gas phase and in solution. International Journal of Quantum Chemistry, 119(2):e25686, 2019.
- [91] Akira Takada, Reinhard Conradt, and Pascal Richet. Partition function and configurational entropy in non-equilibrium states: A new theoretical model. Entropy, 20(4), 2018.
- [92] Georg Brehm, Markus Reiher, Boris Le Guennic, Michael Leibold, Siegfried Schindler, Frank W. Heinemann, and Siegfried Schneider. Investigation of the low-spin to high-spin transition in a novel [Fe(pmea)(NCS)2] complex by IR and raman spectroscopy and DFT calculations. Journal of Raman Spectroscopy, 37(1‐3):108–122, 2006.
- [93] Philipp Pracht and Stefan Grimme. Calculation of absolute molecular entropies and heat capacities made simple. Chem. Sci., 12:6551–6568, 2021.
- [94] D.A. McQuarrie and M.Q.D. A. Statistical Mechanics. Chemistry Series. Harper & Row, 1975.
- [95] T.L. Hill. An Introduction to Statistical Thermodynamics. Addison-Wesley series in chemistry. Dover Publications, 1986.
- [96] Saswata Bhattacharya, Daniel Berger, Karsten Reuter, Luca M. Ghiringhelli, and Sergey V. Levchenko. Theoretical evidence for unexpected O-rich phases at corners of MgO surfaces. Phys. Rev. Materials, 1:071601, Dec 2017.
- [97] Preeti Bhumla, Manish Kumar, and Saswata Bhattacharya. Theoretical insights into C-H bond activation of methane by transition metal clusters: the role of anharmonic effects. Nanoscale Adv., 3:575–583, 2021.
- [98] David Shortle. Propensities, probabilities, and the boltzmann hypothesis. Computational and Theoretical Chemistry, 12:1298–1302, 2003.
- [99] D. Schebarchov, F. Baletto, and D. J. Wales. Structure, thermodynamics, and rearrangement mechanisms in gold clusters—insights from the energy landscapes framework. Nanoscale, 10:2004–2016, 2018.
- [100] Bryan R. Goldsmith, Jacob Florian, Jin-Xun Liu, Philipp Gruene, Jonathan T. Lyon, David M. Rayner, André Fielicke, Matthias Scheffler, and Luca M. Ghiringhelli. Two-to-three dimensional transition in neutral gold clusters: The crucial role of van der waals interactions and temperature. Phys. Rev. Materials, 3:016002, Jan 2019.
- [101] Mukul Kabir, Abhijit Mookerjee, and A. K. Bhattacharya. Structure and stability of copper clusters: A tight-binding molecular dynamics study. Phys. Rev. A, 69:043203, Apr 2004.
- [102] Galip H. Guvelioglu, Pingping Ma, Xiaoyi He, Robert C. Forrey, and Hansong Cheng. First principles studies on the growth of small Cu clusters and the dissociative chemisorption of . Phys. Rev. B, 73:155436, Apr 2006.
- [103] Jianhua Zhang, Cai-Zhuang Wang, Zizhong Zhu, Qing Huo Liu, and Kai-Ming Ho. Multimode Jahn-Teller effect in bulk systems: A case of the center in diamond. Phys. Rev. B, 97:165204, Apr 2018.
- [104] Matija Zlatar, Maja Gruden-Pavlović, Carl-Wilhelm Schläpfer, and Claude Daul. Intrinsic distortion path in the analysis of the Jahn–Teller effect. Journal of Molecular Structure: THEOCHEM, 954(1):86–93, 2010. DFT09, 13th International Conference on the Applications of Density Functional Theory in Chemistry and Physics.
- [105] U. Opik and Maurice Henry Lecorney Pryce. Studies of the Jahn-Teller effect. i. a survey of the static problem. Proceedings of the Royal Society of London. Series A. Mathematical and Physical Sciences, 238(1215):425–447, 1957.
- [106] Stan J. Tinnemans, J. Gerbrand Mesu, Kaisa Kervinen, Tom Visser, T. Alexander Nijhuis, Andrew M. Beale, Daphne E. Keller, Ad M.J. van der Eerden, and Bert M. Weckhuysen. Combining operando techniques in one spectroscopic-reaction cell: New opportunities for elucidating the active site and related reaction mechanism in catalysis. Catalysis Today, 113(1):3–15, 2006. Recent Advances in In situ and Operando Studies of Catalytic Reactions.
- [107] M. Brandhorst, S. Cristol, M. Capron, C. Dujardin, H. Vezin, G. Le bourdon, and E. Payen. Catalytic oxidation of methanol on Mo/Al2O3 catalyst: An EPR and raman/infrared operando spectroscopies study. Catalysis Today, 113(1):34–39, 2006. Recent Advances in In situ and Operando Studies of Catalytic Reactions.
- [108] Kazuki Hashimoto, Venkata Ramaiah Badarla, Akira Kawai, and Takuro Ideguchi. Complementary vibrational spectroscopy. Nature Communications, 10(1):4411, Sep 2019.
- [109] A. Fielicke, G. von Helden, and G. Meijer. Far-infrared spectroscopy of isolated transition metal clusters. The European Physical Journal D - Atomic, Molecular, Optical and Plasma Physics, 34(1):83–88, Jul 2005.
- [110] D. E. Powers, S. G. Hansen, M. E. Geusic, A. C. Puiu, J. B. Hopkins, T. G. Dietz, M. A. Duncan, P. R. R. Langridge-Smith, and R. E. Smalley. Supersonic metal cluster beams: laser photoionization studies of copper cluster (Cu2). The Journal of Physical Chemistry, 86(14):2556–2560, 1982.
- [111] Olga V. Lushchikova, Douwe M. M. Huitema, Pablo López-Tarifa, Lucas Visscher, Zahra Jamshidi, and Joost M. Bakker. Structures of Cu (n = 3-10) clusters obtained by infrared action spectroscopy. The Journal of Physical Chemistry Letters, 10(9):2151–2155, 2019. PMID: 30977666.
- [112] Takeshi Yanai, David P Tew, and Nicholas C Handy. A new hybrid exchange-correlation functional using the coulomb-attenuating method (CAM-B3LYP). Chemical Physics Letters, 393(1):51–57, 2004.
- [113] S. Lecoultre, A. Rydlo, C. Felix, J. Buttet, S. Gilb, and W. Harbich. Optical absorption of small copper clusters in neon: Cun, (n = 1-9). The Journal of Chemical Physics, 134(7):074303, 2011.
- [114] Christoph Sieber, Jean Buttet, Wolfgang Harbich, Christian Félix, Roland Mitrić, and Vlasta Bonači ć Koutecký. Isomer-specific spectroscopy of metal clusters trapped in a matrix: . Phys. Rev. A, 70:041201, Oct 2004.
- [115] Vivike J. F. Lapoutre, Marko Haertelt, Gerard Meijer, André Fielicke, and Joost M. Bakker. Communication: IR spectroscopy of neutral transition metal clusters through thermionic emission. The Journal of Chemical Physics, 139(12):121101, 2013.
- [116] Cesar Castillo-Quevedo, Jose Luis Cabellos, Raul Aceves, Roberto Núñez-González, and Alvaro Posada-Amarillas. Cu-doped KCl unfolded band structure and optical properties studied by DFT calculations. Materials, 13(19), 2020.
- [117] Gerald Geudtner, Patrizia Calaminici, Javier Carmona-Espíndola, Jorge Martín del Campo, Víctor Daniel Domínguez-Soria, Robert Flores Moreno, Gabriel Ulises Gamboa, Annick Goursot, Andreas M. Köster, José Ulises Reveles, Tzonka Mineva, José Manuel Vásquez-Pérez, Alberto Vela, Bernardo Zúñinga Gutierrez, and Dennis R. Salahub. deMon2k. WIREs Computational Molecular Science, 2(4):548–555, 2012.
- [118] Andreas M. Koster, J. Ulises Reveles, and Jorge M. del Campo. Calculation of exchange-correlation potentials with auxiliary function densities. The Journal of Chemical Physics, 121(8):3417–3424, 2004.
Appendix A ZPE descomposition
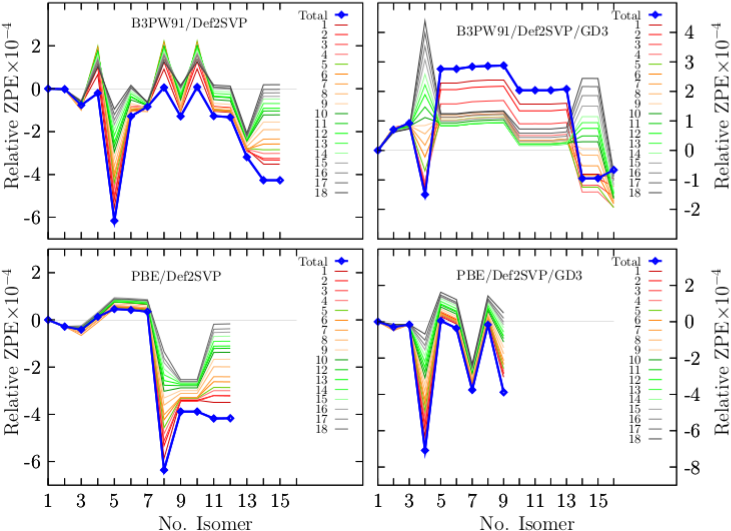
Appendix B IR spectra
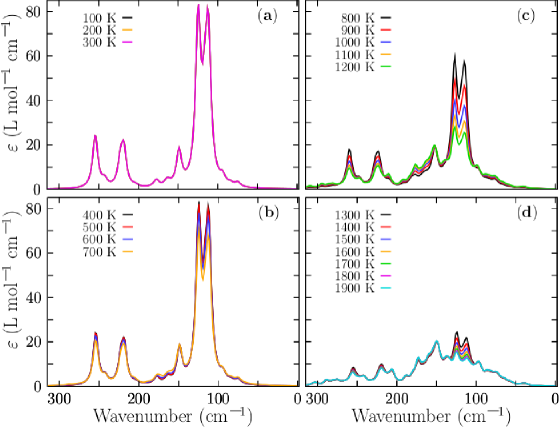
Appendix C UV-Visible spectra for different temperatures
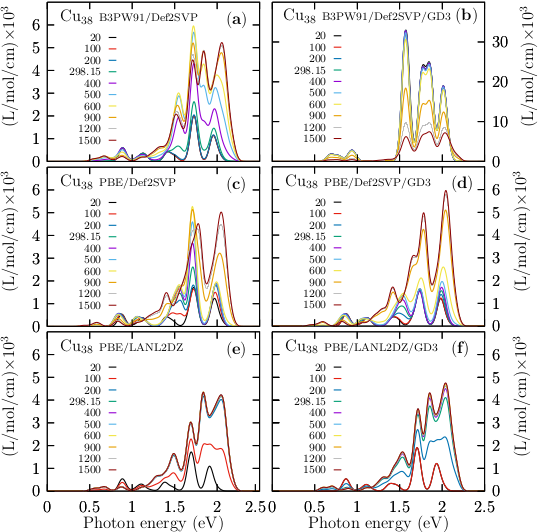
Appendix D Geometry at B3PW91/Def2svp level of theory
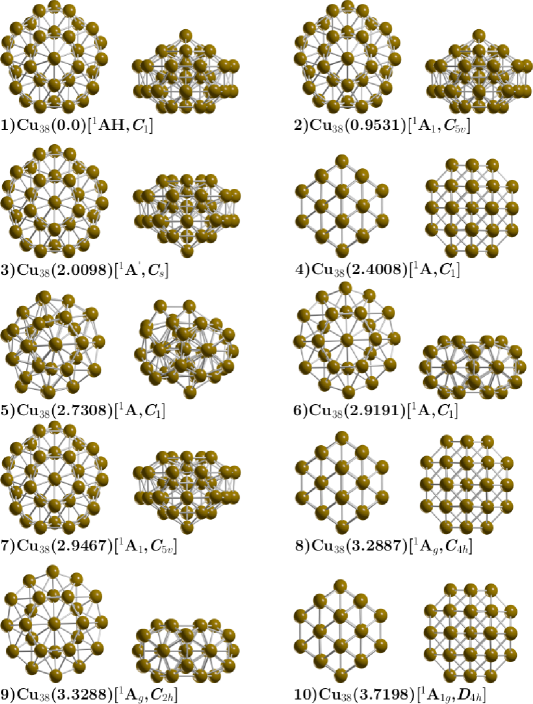
Appendix E XYZ atomic coordiantes
38 0.000000000 gibss_0001.out Cu -1.741167000000 -0.158313000000 3.424442000000 Cu -1.777542000000 -0.161558000000 -0.000026000000 Cu -0.158340000000 1.741115000000 3.424465000000 Cu 0.158346000000 -1.741130000000 -3.424473000000 Cu -1.638340000000 -1.965976000000 -1.760408000000 Cu -1.965973000000 1.638364000000 -1.760399000000 Cu -0.161559000000 1.777542000000 0.000001000000 Cu 1.741184000000 0.158312000000 -3.424443000000 Cu -1.638381000000 -1.965997000000 1.760353000000 Cu 1.965966000000 -1.638365000000 1.760395000000 Cu -0.000022000000 -0.000001000000 1.661034000000 Cu 0.158252000000 -1.741146000000 3.424462000000 Cu 2.092781000000 -3.341499000000 0.000013000000 Cu 3.341505000000 2.092789000000 0.000059000000 Cu 1.777542000000 0.161558000000 0.000020000000 Cu 0.317743000000 -3.496543000000 1.724356000000 Cu 0.317778000000 -3.496524000000 -1.724370000000 Cu -3.496557000000 -0.317767000000 1.724319000000 Cu 3.496520000000 0.317741000000 1.724395000000 Cu -1.966017000000 1.638350000000 1.760362000000 Cu 3.664053000000 -1.455978000000 0.000035000000 Cu 0.161557000000 -1.777536000000 -0.000006000000 Cu -3.341499000000 -2.092782000000 -0.000042000000 Cu -3.664057000000 1.455992000000 -0.000039000000 Cu 3.496552000000 0.317775000000 -1.724315000000 Cu 1.638379000000 1.966002000000 -1.760352000000 Cu 1.741096000000 0.158279000000 3.424485000000 Cu -0.317788000000 3.496524000000 1.724365000000 Cu -1.741081000000 -0.158288000000 -3.424488000000 Cu 1.966013000000 -1.638353000000 -1.760365000000 Cu -0.158253000000 1.741137000000 -3.424459000000 Cu -0.317738000000 3.496539000000 -1.724349000000 Cu -1.455990000000 -3.664048000000 -0.000025000000 Cu 0.000024000000 0.000009000000 -1.661040000000 Cu -2.092798000000 3.341499000000 -0.000013000000 Cu 1.455986000000 3.664055000000 0.000042000000 Cu -3.496517000000 -0.317760000000 -1.724399000000 Cu 1.638342000000 1.965984000000 1.760408000000 38 0.169425003 mol_0002.out Cu 3.513500000000 1.796474000000 0.000001000000 Cu 0.001079000000 1.772700000000 -0.000004000000 Cu 3.516966000000 -0.002124000000 -1.698724000000 Cu -3.516961000000 0.002132000000 1.698727000000 Cu -1.808652000000 1.810631000000 1.758032000000 Cu -1.808655000000 1.810629000000 -1.758028000000 Cu 0.000024000000 -0.000012000000 -1.680316000000 Cu -3.513525000000 -1.796471000000 -0.000010000000 Cu 1.810831000000 1.808461000000 1.758030000000 Cu 1.808648000000 -1.810647000000 1.758046000000 Cu 1.771217000000 -0.001082000000 0.000009000000 Cu 3.516981000000 -0.002120000000 1.698709000000 Cu -0.001045000000 -1.735089000000 3.435940000000 Cu -0.002123000000 -3.498863000000 -1.735691000000 Cu -0.001088000000 -1.772720000000 0.000003000000 Cu 1.769137000000 -0.001066000000 3.418064000000 Cu -1.769051000000 0.001079000000 3.418037000000 Cu 1.765981000000 3.525659000000 -0.000002000000 Cu 1.761732000000 -3.527775000000 0.000020000000 Cu 1.810839000000 1.808464000000 -1.758034000000 Cu -0.002148000000 -3.498870000000 1.735669000000 Cu 0.000016000000 -0.000009000000 1.680314000000 Cu 0.002097000000 3.498861000000 1.735676000000 Cu 0.002097000000 3.498863000000 -1.735698000000 Cu -1.766014000000 -3.525655000000 -0.000018000000 Cu -1.810842000000 -1.808450000000 -1.758047000000 Cu 3.511350000000 -1.800707000000 0.000022000000 Cu 1.769120000000 -0.001061000000 -3.418053000000 Cu -3.511372000000 1.800704000000 0.000010000000 Cu -1.810841000000 -1.808447000000 1.758028000000 Cu -3.516989000000 0.002137000000 -1.698717000000 Cu -1.769072000000 0.001075000000 -3.418030000000 Cu 0.001057000000 1.735091000000 3.435939000000 Cu -1.771212000000 0.001074000000 -0.000010000000 Cu 0.001046000000 1.735085000000 -3.435936000000 Cu -0.001046000000 -1.735083000000 -3.435939000000 Cu -1.761741000000 3.527776000000 0.000002000000 Cu 1.808658000000 -1.810644000000 -1.758021000000 38 1.384892499 mol_0003.out Cu -3.517960000000 1.780496000000 0.000000000000 Cu 0.000000000000 1.785134000000 0.000000000000 Cu -3.510601000000 0.000000000000 1.725845000000 Cu 3.510601000000 0.000000000000 -1.725845000000 Cu 1.809494000000 1.809494000000 -1.759436000000 Cu 1.809494000000 1.809494000000 1.759436000000 Cu 0.000000000000 0.000000000000 1.661000000000 Cu 3.517960000000 -1.780496000000 0.000000000000 Cu -1.809494000000 1.809494000000 -1.759436000000 Cu -1.809494000000 -1.809494000000 -1.759436000000 Cu -1.785134000000 0.000000000000 0.000000000000 Cu -3.510601000000 0.000000000000 -1.725845000000 Cu 0.000000000000 -1.748410000000 -3.423917000000 Cu 0.000000000000 -3.510601000000 1.725845000000 Cu 0.000000000000 -1.785134000000 0.000000000000 Cu -1.748410000000 0.000000000000 -3.423917000000 Cu 1.748410000000 0.000000000000 -3.423917000000 Cu -1.780496000000 3.517960000000 0.000000000000 Cu -1.780496000000 -3.517960000000 0.000000000000 Cu -1.809494000000 1.809494000000 1.759436000000 Cu 0.000000000000 -3.510601000000 -1.725845000000 Cu 0.000000000000 0.000000000000 -1.661000000000 Cu 0.000000000000 3.510601000000 -1.725845000000 Cu 0.000000000000 3.510601000000 1.725845000000 Cu 1.780496000000 -3.517960000000 0.000000000000 Cu 1.809494000000 -1.809494000000 1.759436000000 Cu -3.517960000000 -1.780496000000 0.000000000000 Cu -1.748410000000 0.000000000000 3.423917000000 Cu 3.517960000000 1.780496000000 0.000000000000 Cu 1.809494000000 -1.809494000000 -1.759436000000 Cu 3.510601000000 0.000000000000 1.725845000000 Cu 1.748410000000 0.000000000000 3.423917000000 Cu 0.000000000000 1.748410000000 -3.423917000000 Cu 1.785134000000 0.000000000000 0.000000000000 Cu 0.000000000000 1.748410000000 3.423917000000 Cu 0.000000000000 -1.748410000000 3.423917000000 Cu 1.780496000000 3.517960000000 0.000000000000 Cu -1.809494000000 -1.809494000000 1.759436000000 38 5.798100000 mol_0006.out Cu 1.172939000000 1.097826000000 3.378465000000 Cu -0.032807000000 -2.145198000000 0.000000000000 Cu 2.431734000000 -0.653745000000 2.012704000000 Cu -0.938438000000 -2.463862000000 -3.334080000000 Cu -1.123725000000 -0.000130000000 0.000000000000 Cu 1.173103000000 -2.873666000000 -2.087959000000 Cu 1.172939000000 1.097826000000 -3.378465000000 Cu -0.938631000000 2.409535000000 -3.373250000000 Cu -0.032655000000 -0.662804000000 2.040109000000 Cu -0.032401000000 1.735377000000 1.260561000000 Cu 2.431564000000 1.712147000000 1.243736000000 Cu -0.938609000000 0.033485000000 4.145587000000 Cu -2.182325000000 0.675722000000 2.079899000000 Cu -0.938756000000 3.952969000000 1.249204000000 Cu -0.938756000000 3.952969000000 -1.249204000000 Cu 1.145976000000 -1.308226000000 4.026584000000 Cu -2.182373000000 -1.769544000000 1.285501000000 Cu 1.146150000000 -4.233754000000 0.000000000000 Cu 1.145845000000 3.425285000000 2.488512000000 Cu 1.173103000000 -2.873666000000 2.087959000000 Cu -2.182362000000 2.186891000000 0.000000000000 Cu -3.439847000000 -0.000200000000 0.000000000000 Cu -0.938319000000 -3.932561000000 1.312904000000 Cu 2.431736000000 -2.116158000000 0.000000000000 Cu 1.145845000000 3.425285000000 -2.488512000000 Cu -0.032401000000 1.735377000000 -1.260561000000 Cu -0.938631000000 2.409535000000 3.373250000000 Cu 1.270655000000 -0.000203000000 0.000000000000 Cu 1.145976000000 -1.308226000000 -4.026584000000 Cu -2.182373000000 -1.769544000000 -1.285501000000 Cu -0.938609000000 0.033485000000 -4.145587000000 Cu -0.032655000000 -0.662804000000 -2.040109000000 Cu -0.938438000000 -2.463862000000 3.334080000000 Cu -2.182325000000 0.675722000000 -2.079899000000 Cu 2.431734000000 -0.653745000000 -2.012704000000 Cu 1.172889000000 3.552872000000 0.000000000000 Cu -0.938319000000 -3.932561000000 -1.312904000000 Cu 2.431564000000 1.712147000000 -1.243736000000 38 5.798100000 mol_0005.out Cu 1.105608000000 3.375910000000 -1.172987000000 Cu -2.145190000000 0.004974000000 0.032902000000 Cu -0.649183000000 2.014217000000 -2.431704000000 Cu -2.471547000000 -3.328357000000 0.938545000000 Cu -0.000079000000 -0.000001000000 1.123724000000 Cu -2.878554000000 -2.081286000000 -1.172976000000 Cu 1.089932000000 -3.381006000000 -1.172979000000 Cu 2.401746000000 -3.378831000000 0.938519000000 Cu -0.658068000000 2.041641000000 0.032685000000 Cu 1.738298000000 1.256532000000 0.032323000000 Cu 1.714919000000 1.239763000000 -2.431641000000 Cu 0.043144000000 4.145497000000 0.938610000000 Cu 0.680643000000 2.078324000000 2.182295000000 Cu 3.955899000000 1.240030000000 0.938578000000 Cu 3.950099000000 -1.258371000000 0.938578000000 Cu -1.298933000000 4.029609000000 -1.145915000000 Cu -1.766459000000 1.289600000000 2.182453000000 Cu -4.233794000000 0.009824000000 -1.145961000000 Cu 3.430997000000 2.480561000000 -1.145998000000 Cu -2.868866000000 2.094620000000 -1.172981000000 Cu 2.186984000000 -0.005073000000 2.182263000000 Cu -0.000045000000 0.000000000000 3.439846000000 Cu -3.929462000000 1.322022000000 0.938496000000 Cu -2.116261000000 0.004912000000 -2.431642000000 Cu 3.419450000000 -2.496451000000 -1.145994000000 Cu 1.732447000000 -1.264583000000 0.032321000000 Cu 2.417399000000 3.367646000000 0.938517000000 Cu -0.000260000000 0.000001000000 -1.270656000000 Cu -1.317613000000 -4.023537000000 -1.145918000000 Cu -1.772420000000 -1.281396000000 2.182451000000 Cu 0.023909000000 -4.145655000000 0.938616000000 Cu -0.667531000000 -2.038564000000 0.032684000000 Cu -2.456078000000 3.339789000000 0.938548000000 Cu 0.670994000000 -2.081457000000 2.182292000000 Cu -0.658520000000 -2.011176000000 -2.431704000000 Cu 3.552810000000 -0.008241000000 -1.173050000000 Cu -3.935562000000 -1.303783000000 0.938496000000 Cu 1.709147000000 -1.247704000000 -2.431634000000 38 5.810022499 mol_0007.out Cu 1.766398000000 -3.082086000000 -1.172799000000 Cu 1.440661000000 1.589530000000 0.032911000000 Cu 1.930492000000 -0.866814000000 -2.431711000000 Cu -0.816402000000 4.064729000000 0.938172000000 Cu -0.000031000000 0.000142000000 1.123925000000 Cu 0.382489000000 3.531342000000 -1.172949000000 Cu -3.240376000000 1.454978000000 -1.172933000000 Cu -4.118083000000 0.479809000000 0.938149000000 Cu 1.956875000000 -0.878715000000 0.032801000000 Cu -0.231030000000 -2.132524000000 0.032845000000 Cu -0.227732000000 -2.103731000000 -2.431572000000 Cu 3.049608000000 -2.808643000000 0.938449000000 Cu 1.087887000000 -1.897327000000 2.182398000000 Cu -1.728917000000 -3.768022000000 0.938368000000 Cu -3.580249000000 -2.090260000000 0.938549000000 Cu 3.862306000000 -1.734288000000 -1.146249000000 Cu 2.140799000000 0.448442000000 2.182400000000 Cu 2.842814000000 3.137229000000 -1.146425000000 Cu -0.455870000000 -4.208980000000 -1.146341000000 Cu 3.477118000000 0.727378000000 -1.172831000000 Cu -1.468555000000 -1.620845000000 2.182378000000 Cu -0.000120000000 0.000172000000 3.440164000000 Cu 3.613691000000 2.032444000000 0.938308000000 Cu 1.420985000000 1.567972000000 -2.431672000000 Cu -4.143913000000 -0.867104000000 -1.146419000000 Cu -2.099748000000 -0.439303000000 0.032870000000 Cu 0.881639000000 -4.050719000000 0.938679000000 Cu -0.000046000000 -0.000099000000 -1.269991000000 Cu -2.105204000000 3.672841000000 -1.146580000000 Cu 0.235173000000 2.174649000000 2.182401000000 Cu -3.094383000000 2.758837000000 0.938321000000 Cu -1.066643000000 1.861156000000 0.032997000000 Cu 4.125010000000 -0.413264000000 0.938640000000 Cu -1.995549000000 0.895815000000 2.182347000000 Cu -1.052144000000 1.835710000000 -2.431642000000 Cu -2.385389000000 -2.632250000000 -1.172838000000 Cu 1.667604000000 3.795290000000 0.938410000000 Cu -2.071166000000 -0.433489000000 -2.431532000000 38 6.768842500 mol_0008.out Cu 3.378495000000 -1.097740000000 -1.172970000000 Cu 0.000000000000 2.145197000000 0.032772000000 Cu 2.012643000000 0.653947000000 -2.431766000000 Cu -3.334103000000 2.463807000000 0.938557000000 Cu 0.000000000000 0.000000000000 1.123689000000 Cu -2.088025000000 2.873919000000 -1.172970000000 Cu -3.378495000000 -1.097740000000 -1.172970000000 Cu -3.373514000000 -2.409562000000 0.938557000000 Cu 2.040204000000 0.662902000000 0.032772000000 Cu 1.260915000000 -1.735501000000 0.032772000000 Cu 1.243882000000 -1.712056000000 -2.431766000000 Cu 4.145535000000 -0.033525000000 0.938557000000 Cu 2.079873000000 -0.675792000000 2.182292000000 Cu 1.249157000000 -3.952998000000 0.938557000000 Cu -1.249157000000 -3.952998000000 0.938557000000 Cu 4.026559000000 1.308308000000 -1.146004000000 Cu 1.285432000000 1.769246000000 2.182292000000 Cu 0.000000000000 4.233775000000 -1.146004000000 Cu 2.488551000000 -3.425196000000 -1.146004000000 Cu 2.088025000000 2.873919000000 -1.172970000000 Cu 0.000000000000 -2.186908000000 2.182292000000 Cu 0.000000000000 0.000000000000 3.439811000000 Cu 1.312925000000 3.932278000000 0.938557000000 Cu 0.000000000000 2.116218000000 -2.431766000000 Cu -2.488551000000 -3.425196000000 -1.146004000000 Cu -1.260915000000 -1.735501000000 0.032772000000 Cu 3.373514000000 -2.409562000000 0.938557000000 Cu 0.000000000000 0.000000000000 -1.270691000000 Cu -4.026559000000 1.308308000000 -1.146004000000 Cu -1.285432000000 1.769246000000 2.182292000000 Cu -4.145535000000 -0.033525000000 0.938557000000 Cu -2.040204000000 0.662902000000 0.032772000000 Cu 3.334103000000 2.463807000000 0.938557000000 Cu -2.079873000000 -0.675792000000 2.182292000000 Cu -2.012643000000 0.653947000000 -2.431766000000 Cu 0.000000000000 -3.552360000000 -1.172970000000 Cu -1.312925000000 3.932278000000 0.938557000000 Cu -1.243882000000 -1.712056000000 -2.431766000000 38 6.771352500 mol_0009.out Cu 3.378495000000 -1.097740000000 -1.172928000000 Cu 0.000000000000 2.145197000000 0.032814000000 Cu 2.012643000000 0.653947000000 -2.431724000000 Cu -3.334082000000 2.463861000000 0.938439000000 Cu 0.000000000000 0.000000000000 1.123731000000 Cu -2.088025000000 2.873919000000 -1.172928000000 Cu -3.378495000000 -1.097740000000 -1.172928000000 Cu -3.373559000000 -2.409526000000 0.938439000000 Cu 2.040204000000 0.662902000000 0.032814000000 Cu 1.260915000000 -1.735501000000 0.032814000000 Cu 1.243882000000 -1.712056000000 -2.431724000000 Cu 4.145550000000 -0.033581000000 0.938439000000 Cu 2.079873000000 -0.675792000000 2.182334000000 Cu 1.249108000000 -3.953030000000 0.938439000000 Cu -1.249108000000 -3.953030000000 0.938439000000 Cu 4.026559000000 1.308308000000 -1.145962000000 Cu 1.285432000000 1.769246000000 2.182334000000 Cu 0.000000000000 4.233775000000 -1.145962000000 Cu 2.488551000000 -3.425196000000 -1.145962000000 Cu 2.088025000000 2.873919000000 -1.172928000000 Cu 0.000000000000 -2.186908000000 2.182334000000 Cu 0.000000000000 0.000000000000 3.439853000000 Cu 1.312983000000 3.932276000000 0.938439000000 Cu 0.000000000000 2.116218000000 -2.431724000000 Cu -2.488551000000 -3.425196000000 -1.145962000000 Cu -1.260915000000 -1.735501000000 0.032814000000 Cu 3.373559000000 -2.409526000000 0.938439000000 Cu 0.000000000000 0.000000000000 -1.270649000000 Cu -4.026559000000 1.308308000000 -1.145962000000 Cu -1.285432000000 1.769246000000 2.182334000000 Cu -4.145550000000 -0.033581000000 0.938439000000 Cu -2.040204000000 0.662902000000 0.032814000000 Cu 3.334082000000 2.463861000000 0.938439000000 Cu -2.079873000000 -0.675792000000 2.182334000000 Cu -2.012643000000 0.653947000000 -2.431724000000 Cu 0.000000000000 -3.552360000000 -1.172928000000 Cu -1.312983000000 3.932276000000 0.938439000000 Cu -1.243882000000 -1.712056000000 -2.431724000000 38 8.858417499 mol_0012.out Cu -2.222929000000 -0.666563000000 2.051042000000 Cu 1.100741000000 0.048218000000 -4.162907000000 Cu 1.100741000000 0.048218000000 4.162907000000 Cu 0.236184000000 -2.141805000000 0.000000000000 Cu 0.236315000000 1.732655000000 1.258584000000 Cu -0.955762000000 -1.309398000000 -4.030462000000 Cu 1.100862000000 3.974155000000 1.240609000000 Cu 1.100619000000 -3.944546000000 1.332148000000 Cu 2.353877000000 -1.770962000000 1.286346000000 Cu 2.354134000000 2.188481000000 0.000000000000 Cu -2.222929000000 -0.666563000000 -2.051042000000 Cu 2.353995000000 0.676142000000 -2.081502000000 Cu 2.353995000000 0.676142000000 2.081502000000 Cu 2.353877000000 -1.770962000000 -1.286346000000 Cu -1.009819000000 1.103123000000 3.394227000000 Cu -1.009794000000 3.569354000000 0.000000000000 Cu 0.236445000000 -0.661760000000 -2.036789000000 Cu -2.223003000000 1.744823000000 1.267637000000 Cu -2.223003000000 1.744823000000 -1.267637000000 Cu -0.955915000000 -4.237971000000 0.000000000000 Cu 1.100862000000 3.974155000000 -1.240609000000 Cu -0.955746000000 3.428780000000 2.490848000000 Cu -0.955762000000 -1.309398000000 4.030462000000 Cu -3.425186000000 0.000016000000 0.000000000000 Cu -0.955746000000 3.428780000000 -2.490848000000 Cu -1.019001000000 -0.000005000000 0.000000000000 Cu 1.100619000000 -3.944546000000 -1.332148000000 Cu -1.009814000000 -2.887484000000 2.098006000000 Cu -1.009819000000 1.103123000000 -3.394227000000 Cu 1.100737000000 2.408075000000 3.395964000000 Cu 1.100737000000 2.408075000000 -3.395964000000 Cu 1.100752000000 -2.485827000000 3.339602000000 Cu -1.009814000000 -2.887484000000 -2.098006000000 Cu 1.100752000000 -2.485827000000 -3.339602000000 Cu 0.236445000000 -0.661760000000 2.036789000000 Cu 0.236315000000 1.732655000000 -1.258584000000 Cu -2.223136000000 -2.156773000000 0.000000000000 Cu 1.428174000000 -0.000159000000 0.000000000000 38 8.858417499 mol_0010.out Cu -2.222929000000 -0.666563000000 2.051042000000 Cu 1.100741000000 0.048218000000 -4.162907000000 Cu 1.100741000000 0.048218000000 4.162907000000 Cu 0.236184000000 -2.141805000000 0.000000000000 Cu 0.236315000000 1.732655000000 1.258584000000 Cu -0.955762000000 -1.309398000000 -4.030462000000 Cu 1.100862000000 3.974155000000 1.240609000000 Cu 1.100619000000 -3.944546000000 1.332148000000 Cu 2.353877000000 -1.770962000000 1.286346000000 Cu 2.354134000000 2.188481000000 0.000000000000 Cu -2.222929000000 -0.666563000000 -2.051042000000 Cu 2.353995000000 0.676142000000 -2.081502000000 Cu 2.353995000000 0.676142000000 2.081502000000 Cu 2.353877000000 -1.770962000000 -1.286346000000 Cu -1.009819000000 1.103123000000 3.394227000000 Cu -1.009794000000 3.569354000000 0.000000000000 Cu 0.236445000000 -0.661760000000 -2.036789000000 Cu -2.223003000000 1.744823000000 1.267637000000 Cu -2.223003000000 1.744823000000 -1.267637000000 Cu -0.955915000000 -4.237971000000 0.000000000000 Cu 1.100862000000 3.974155000000 -1.240609000000 Cu -0.955746000000 3.428780000000 2.490848000000 Cu -0.955762000000 -1.309398000000 4.030462000000 Cu -3.425186000000 0.000016000000 0.000000000000 Cu -0.955746000000 3.428780000000 -2.490848000000 Cu -1.019001000000 -0.000005000000 0.000000000000 Cu 1.100619000000 -3.944546000000 -1.332148000000 Cu -1.009814000000 -2.887484000000 2.098006000000 Cu -1.009819000000 1.103123000000 -3.394227000000 Cu 1.100737000000 2.408075000000 3.395964000000 Cu 1.100737000000 2.408075000000 -3.395964000000 Cu 1.100752000000 -2.485827000000 3.339602000000 Cu -1.009814000000 -2.887484000000 -2.098006000000 Cu 1.100752000000 -2.485827000000 -3.339602000000 Cu 0.236445000000 -0.661760000000 2.036789000000 Cu 0.236315000000 1.732655000000 -1.258584000000 Cu -2.223136000000 -2.156773000000 0.000000000000 Cu 1.428174000000 -0.000159000000 0.000000000000 38 8.858417499 mol_0011.out Cu 0.672221000000 -2.049247000000 2.223083000000 Cu -0.059920000000 4.162819000000 -1.100824000000 Cu -0.036451000000 -4.163096000000 -1.100801000000 Cu 2.141760000000 0.006043000000 -0.236583000000 Cu -1.729069000000 -1.263491000000 -0.236235000000 Cu 1.298094000000 4.034150000000 0.955790000000 Cu -3.970563000000 -1.251894000000 -1.100768000000 Cu 3.948301000000 -1.320963000000 -1.100682000000 Cu 1.774377000000 -1.281426000000 -2.354043000000 Cu -2.188620000000 -0.006173000000 -2.354039000000 Cu 0.660641000000 2.053007000000 2.223109000000 Cu -0.682173000000 2.079574000000 -2.354024000000 Cu -0.670447000000 -2.083396000000 -2.354016000000 Cu 1.767129000000 1.291436000000 -2.354026000000 Cu -1.093350000000 -3.397286000000 1.009804000000 Cu -3.569419000000 -0.010064000000 1.009902000000 Cu 0.656027000000 2.038749000000 -0.236504000000 Cu -1.741143000000 -1.272370000000 2.223069000000 Cu -1.748290000000 1.262528000000 2.223051000000 Cu 4.237851000000 0.011942000000 0.955925000000 Cu -3.977546000000 1.229473000000 -1.100771000000 Cu -3.421480000000 -2.500517000000 0.955912000000 Cu 1.320844000000 -4.026777000000 0.955790000000 Cu 0.000128000000 -0.000004000000 3.425264000000 Cu -3.435520000000 2.481187000000 0.955890000000 Cu 0.000233000000 0.000006000000 1.018926000000 Cu 3.940814000000 1.343224000000 -1.100653000000 Cu 2.893107000000 -2.089651000000 1.009758000000 Cu -1.112498000000 3.391098000000 1.009796000000 Cu -2.398514000000 -3.402684000000 -1.100749000000 Cu -2.417655000000 3.389102000000 -1.100768000000 Cu 2.495270000000 -3.332592000000 -1.100784000000 Cu 2.881258000000 2.105925000000 1.009769000000 Cu 2.476438000000 3.346598000000 -1.100752000000 Cu 0.667537000000 -2.035027000000 -0.236513000000 Cu -1.736160000000 1.253722000000 -0.236234000000 Cu 2.156758000000 0.006076000000 2.223139000000 Cu 0.000029000000 -0.000002000000 -1.428204000000 38 9.817865000 mol_0013.out Cu 2.051086000000 0.666438000000 2.222906000000 Cu -4.162907000000 -0.048223000000 -1.100760000000 Cu 4.162907000000 -0.048223000000 -1.100760000000 Cu 0.000000000000 2.141804000000 -0.236213000000 Cu 1.258921000000 -1.732756000000 -0.236213000000 Cu -4.030411000000 1.309560000000 0.955736000000 Cu 1.240546000000 -3.974062000000 -1.100760000000 Cu 1.332272000000 3.944258000000 -1.100760000000 Cu 1.286559000000 1.770796000000 -2.353905000000 Cu 0.000000000000 -2.188825000000 -2.353905000000 Cu -2.051086000000 0.666438000000 2.222906000000 Cu -2.081696000000 -0.676384000000 -2.353905000000 Cu 2.081696000000 -0.676384000000 -2.353905000000 Cu -1.286559000000 1.770796000000 -2.353905000000 Cu 3.394305000000 -1.102877000000 1.009805000000 Cu 0.000000000000 -3.568984000000 1.009805000000 Cu -2.036977000000 0.661854000000 -0.236213000000 Cu 1.267641000000 -1.744758000000 2.222906000000 Cu -1.267641000000 -1.744758000000 2.222906000000 Cu 0.000000000000 4.237825000000 0.955736000000 Cu -1.240546000000 -3.974062000000 -1.100760000000 Cu 2.490931000000 -3.428472000000 0.955736000000 Cu 4.030411000000 1.309560000000 0.955736000000 Cu 0.000000000000 0.000000000000 3.425167000000 Cu -2.490931000000 -3.428472000000 0.955736000000 Cu 0.000000000000 0.000000000000 1.018982000000 Cu -1.332272000000 3.944258000000 -1.100760000000 Cu 2.097796000000 2.887369000000 1.009805000000 Cu -3.394305000000 -1.102877000000 1.009805000000 Cu 3.396207000000 -2.407882000000 -1.100760000000 Cu -3.396207000000 -2.407882000000 -1.100760000000 Cu 3.339518000000 2.485909000000 -1.100760000000 Cu -2.097796000000 2.887369000000 1.009805000000 Cu -3.339518000000 2.485909000000 -1.100760000000 Cu 2.036977000000 0.661854000000 -0.236213000000 Cu -1.258921000000 -1.732756000000 -0.236213000000 Cu 0.000000000000 2.156639000000 2.222906000000 Cu 0.000000000000 0.000000000000 -1.428193000000 38 12.608357499 mol_0014.out Cu 0.490434000000 0.660951000000 1.214726000000 Cu 0.041111000000 -2.415770000000 -4.048698000000 Cu 2.747884000000 -0.456741000000 1.297885000000 Cu -0.957160000000 4.432673000000 0.000000000000 Cu 2.933411000000 -2.548900000000 0.000000000000 Cu -1.194825000000 -0.252714000000 4.080055000000 Cu 0.041111000000 -2.415770000000 4.048698000000 Cu 0.805645000000 -1.392475000000 0.000000000000 Cu -3.117346000000 -1.304844000000 1.303217000000 Cu -1.764792000000 3.196993000000 -2.011399000000 Cu -3.407687000000 0.940158000000 0.000000000000 Cu -0.655918000000 -3.746245000000 -2.060681000000 Cu 2.339459000000 1.705216000000 -2.439606000000 Cu 2.575996000000 3.985858000000 0.000000000000 Cu -0.655918000000 -3.746245000000 2.060681000000 Cu -3.117346000000 -1.304844000000 -1.303217000000 Cu -1.194825000000 -0.252714000000 -4.080055000000 Cu 2.339459000000 1.705216000000 2.439606000000 Cu -1.764792000000 3.196993000000 2.011399000000 Cu -2.043536000000 0.789376000000 2.053256000000 Cu -1.485285000000 -2.694813000000 0.000000000000 Cu -2.043536000000 0.789376000000 -2.053256000000 Cu 1.222736000000 -0.357953000000 3.292161000000 Cu -0.747423000000 -1.384082000000 2.044147000000 Cu -0.084691000000 1.788565000000 -3.216016000000 Cu -1.353109000000 -0.316455000000 0.000000000000 Cu 0.697615000000 3.101250000000 -1.248349000000 Cu 1.222736000000 -0.357953000000 -3.292161000000 Cu -0.747423000000 -1.384082000000 -2.044147000000 Cu 0.739459000000 -3.761653000000 0.000000000000 Cu 0.490434000000 0.660951000000 -1.214726000000 Cu 0.697615000000 3.101250000000 1.248349000000 Cu 1.530052000000 -2.495519000000 2.066516000000 Cu 2.747884000000 -0.456741000000 -1.297885000000 Cu -1.219515000000 2.063010000000 0.000000000000 Cu 2.446727000000 1.635631000000 0.000000000000 Cu 1.530052000000 -2.495519000000 -2.066516000000 Cu -0.084691000000 1.788565000000 3.216016000000 38 12.610240000 mol_0015.out Cu 0.661737000000 1.214816000000 -0.489643000000 Cu -2.416104000000 -4.048387000000 -0.041442000000 Cu -0.456358000000 1.298349000000 -2.748137000000 Cu 4.432638000000 -0.000416000000 0.958471000000 Cu -2.548506000000 0.000224000000 -2.933904000000 Cu -0.252792000000 4.079621000000 1.195042000000 Cu -2.415364000000 4.048809000000 -0.041520000000 Cu -1.391187000000 0.000096000000 -0.806808000000 Cu -1.305441000000 1.303821000000 3.116816000000 Cu 3.197018000000 -2.012629000000 1.763841000000 Cu 0.938092000000 -0.000081000000 3.408446000000 Cu -3.746590000000 -2.060553000000 0.656123000000 Cu 1.705471000000 -2.439612000000 -2.339240000000 Cu 3.985098000000 0.000031000000 -2.576171000000 Cu -3.746221000000 2.061255000000 0.656151000000 Cu -1.305627000000 -1.303604000000 3.116823000000 Cu -0.253465000000 -4.079581000000 1.195022000000 Cu 1.706049000000 2.439159000000 -2.339213000000 Cu 3.197322000000 2.011978000000 1.764034000000 Cu 0.789643000000 2.053007000000 2.043521000000 Cu -2.694987000000 0.000241000000 1.485112000000 Cu 0.789325000000 -2.053163000000 2.043506000000 Cu -0.357537000000 3.291929000000 -1.222920000000 Cu -1.384112000000 2.043938000000 0.746749000000 Cu 1.788167000000 -3.216935000000 0.084344000000 Cu -0.316686000000 0.000016000000 1.353009000000 Cu 3.101455000000 -1.248427000000 -0.697085000000 Cu -0.358194000000 -3.291889000000 -1.222891000000 Cu -1.384471000000 -2.043712000000 0.746778000000 Cu -3.760542000000 0.000364000000 -0.739288000000 Cu 0.661538000000 -1.214962000000 -0.489649000000 Cu 3.101656000000 1.247976000000 -0.696854000000 Cu -2.495493000000 2.066527000000 -1.530228000000 Cu -0.456629000000 -1.298300000000 -2.748144000000 Cu 2.063161000000 -0.000218000000 1.221068000000 Cu 1.635141000000 -0.000201000000 -2.445930000000 Cu -2.495903000000 -2.066099000000 -1.530218000000 Cu 1.788697000000 3.216615000000 0.084429000000 38 14.035292499 mol_0016.out Cu -1.052256000000 1.044878000000 3.371536000000 Cu -1.616187000000 -0.195146000000 -1.252523000000 Cu -1.816706000000 2.262978000000 1.271817000000 Cu 0.168498000000 -1.011714000000 -4.136016000000 Cu 0.190008000000 -1.181809000000 0.000000000000 Cu -1.052256000000 1.044878000000 -3.371536000000 Cu 2.883659000000 1.294164000000 -2.050286000000 Cu 4.073968000000 -0.704254000000 -1.243667000000 Cu -1.616187000000 -0.195146000000 1.252523000000 Cu 0.767182000000 -0.057525000000 2.037591000000 Cu -0.135534000000 3.590639000000 0.000000000000 Cu -2.204238000000 -1.146248000000 3.351462000000 Cu 0.168498000000 -1.011714000000 4.136016000000 Cu 2.644228000000 -0.831851000000 3.314148000000 Cu 2.031818000000 -2.125272000000 1.274870000000 Cu -3.398127000000 0.846340000000 2.499066000000 Cu -1.910493000000 -2.392712000000 0.000000000000 Cu -3.398127000000 0.846340000000 -2.499066000000 Cu 0.601856000000 2.415250000000 2.056156000000 Cu -3.522549000000 0.909687000000 0.000000000000 Cu 0.364766000000 -3.506967000000 0.000000000000 Cu -0.384193000000 -2.311678000000 2.073765000000 Cu -3.753807000000 -1.254323000000 -1.247595000000 Cu -1.816706000000 2.262978000000 -1.271817000000 Cu 4.073968000000 -0.704254000000 1.243667000000 Cu 2.258432000000 0.103875000000 0.000000000000 Cu 1.357355000000 1.162590000000 4.031350000000 Cu 0.017510000000 1.196017000000 0.000000000000 Cu 1.357355000000 1.162590000000 -4.031350000000 Cu -0.384193000000 -2.311678000000 -2.073765000000 Cu 2.644228000000 -0.831851000000 -3.314148000000 Cu 0.767182000000 -0.057525000000 -2.037591000000 Cu -3.753807000000 -1.254323000000 1.247595000000 Cu 2.031818000000 -2.125272000000 -1.274870000000 Cu 0.601856000000 2.415250000000 -2.056156000000 Cu 2.883659000000 1.294164000000 2.050286000000 Cu -2.204238000000 -1.146248000000 -3.351462000000 Cu 2.131754000000 2.504889000000 0.000000000000
Appendix F XYZ atomic coordiantes at B3PW91/def2-SVP level of theory
38 0.000000000 B3PW91/def2-SVP_0000.out Cu -2.963703000000 -2.066066000000 -1.176713000000 Cu 1.757807000000 -1.330766000000 0.021325000000 Cu -0.711140000000 -2.052754000000 -2.441671000000 Cu 4.039978000000 1.183631000000 0.944558000000 Cu 0.000004000000 0.000034000000 1.145773000000 Cu 3.612560000000 -0.070443000000 -1.176119000000 Cu 1.183124000000 3.412971000000 -1.176385000000 Cu 0.122815000000 4.207722000000 0.944169000000 Cu -0.722457000000 -2.083182000000 0.021582000000 Cu -2.204776000000 0.043067000000 0.022032000000 Cu -2.172348000000 0.042371000000 -2.441606000000 Cu -2.572864000000 -3.331911000000 0.944292000000 Cu -1.829251000000 -1.276275000000 2.190085000000 Cu -3.963903000000 1.417308000000 0.944229000000 Cu -2.440488000000 3.429228000000 0.944660000000 Cu -1.416260000000 -4.086208000000 -1.143821000000 Cu 0.648543000000 -2.133984000000 2.189984000000 Cu 3.448655000000 -2.609514000000 -1.143636000000 Cu -4.323915000000 0.084367000000 -1.143625000000 Cu 1.049369000000 -3.457516000000 -1.176327000000 Cu -1.779112000000 1.345191000000 2.190164000000 Cu 0.000033000000 -0.000036000000 3.480514000000 Cu 2.374105000000 -3.476227000000 0.944588000000 Cu 1.732657000000 -1.310914000000 -2.441600000000 Cu -1.256013000000 4.138099000000 -1.143594000000 Cu -0.640408000000 2.109993000000 0.022042000000 Cu -4.015979000000 -1.261335000000 0.944683000000 Cu -0.000069000000 0.000420000000 -1.322404000000 Cu 3.547588000000 2.473400000000 -1.143439000000 Cu 2.229919000000 -0.042597000000 2.189882000000 Cu 2.507004000000 3.380520000000 0.944835000000 Cu 1.809145000000 1.260635000000 0.021665000000 Cu -0.041393000000 -4.208649000000 0.944935000000 Cu 0.729651000000 2.107616000000 2.189866000000 Cu 1.782640000000 1.242423000000 -2.441696000000 Cu -2.880407000000 2.179969000000 -1.176639000000 Cu 3.989654000000 -1.339820000000 0.945070000000 Cu -0.630762000000 2.079232000000 -2.441656000000 38 0.953172499 B3PW91/def2-SVP_0001.out Cu 3.435954000000 -1.116409000000 -1.176616000000 Cu 0.000000000000 2.204727000000 0.021459000000 Cu 2.066134000000 0.671328000000 -2.441553000000 Cu -3.381685000000 2.507302000000 0.944688000000 Cu 0.000000000000 0.000000000000 1.145877000000 Cu -2.123536000000 2.922797000000 -1.176616000000 Cu -3.435954000000 -1.116409000000 -1.176616000000 Cu -3.429584000000 -2.441374000000 0.944688000000 Cu 2.096820000000 0.681298000000 0.021459000000 Cu 1.295906000000 -1.783662000000 0.021459000000 Cu 1.276941000000 -1.757558000000 -2.441553000000 Cu 4.209596000000 -0.040746000000 0.944688000000 Cu 2.121315000000 -0.689257000000 2.190185000000 Cu 1.262085000000 -4.016154000000 0.944688000000 Cu -1.262085000000 -4.016154000000 0.944688000000 Cu 4.113026000000 1.336403000000 -1.143689000000 Cu 1.311045000000 1.804499000000 2.190185000000 Cu 0.000000000000 4.324691000000 -1.143689000000 Cu 2.541990000000 -3.498749000000 -1.143689000000 Cu 2.123536000000 2.922797000000 -1.176616000000 Cu 0.000000000000 -2.230483000000 2.190185000000 Cu 0.000000000000 0.000000000000 3.480618000000 Cu 1.339588000000 3.990972000000 0.944688000000 Cu 0.000000000000 2.172462000000 -2.441553000000 Cu -2.541990000000 -3.498749000000 -1.143689000000 Cu -1.295906000000 -1.783662000000 0.021459000000 Cu 3.429584000000 -2.441374000000 0.944688000000 Cu 0.000000000000 0.000000000000 -1.322300000000 Cu -4.113026000000 1.336403000000 -1.143689000000 Cu -1.311045000000 1.804499000000 2.190185000000 Cu -4.209596000000 -0.040746000000 0.944688000000 Cu -2.096820000000 0.681298000000 0.021459000000 Cu 3.381685000000 2.507302000000 0.944688000000 Cu -2.121315000000 -0.689257000000 2.190185000000 Cu -2.066134000000 0.671328000000 -2.441553000000 Cu 0.000000000000 -3.612776000000 -1.176616000000 Cu -1.339588000000 3.990972000000 0.944688000000 Cu -1.276941000000 -1.757558000000 -2.441553000000 38 2.009882500 B3PW91/def2-SVP_0002.out Cu 2.235081000000 -0.677404000000 2.084444000000 Cu -1.102557000000 0.054900000000 -4.238071000000 Cu -1.102557000000 0.054900000000 4.238071000000 Cu -0.244412000000 -2.205396000000 0.000000000000 Cu -0.244494000000 1.784050000000 1.295935000000 Cu 0.961756000000 -1.334901000000 -4.108647000000 Cu -1.102733000000 4.047768000000 1.257506000000 Cu -1.102534000000 -4.014031000000 1.361705000000 Cu -2.355422000000 -1.827638000000 1.327480000000 Cu -2.355609000000 2.258764000000 0.000000000000 Cu 2.235081000000 -0.677404000000 -2.084444000000 Cu -2.355582000000 0.697801000000 -2.148195000000 Cu -2.355582000000 0.697801000000 2.148195000000 Cu -2.355422000000 -1.827638000000 -1.327480000000 Cu 1.005985000000 1.119699000000 3.445411000000 Cu 1.005955000000 3.623303000000 0.000000000000 Cu -0.244573000000 -0.681387000000 -2.097194000000 Cu 2.235103000000 1.773224000000 1.288238000000 Cu 2.235103000000 1.773224000000 -1.288238000000 Cu 0.961803000000 -4.320324000000 0.000000000000 Cu -1.102733000000 4.047768000000 -1.257506000000 Cu 0.961741000000 3.495229000000 2.539184000000 Cu 0.961756000000 -1.334901000000 4.108647000000 Cu 3.472040000000 0.000089000000 0.000000000000 Cu 0.961741000000 3.495229000000 -2.539184000000 Cu 1.035302000000 0.000003000000 0.000000000000 Cu -1.102534000000 -4.014031000000 -1.361705000000 Cu 1.005874000000 -2.931073000000 2.129625000000 Cu 1.005985000000 1.119699000000 -3.445411000000 Cu -1.102689000000 2.446869000000 3.460671000000 Cu -1.102689000000 2.446869000000 -3.460671000000 Cu -1.102634000000 -2.535335000000 3.396393000000 Cu 1.005874000000 -2.931073000000 -2.129625000000 Cu -1.102634000000 -2.535335000000 -3.396393000000 Cu -0.244573000000 -0.681387000000 2.097194000000 Cu -0.244494000000 1.784050000000 -1.295935000000 Cu 2.235223000000 -2.191881000000 0.000000000000 Cu -1.494949000000 -0.000098000000 0.000000000000 38 2.400815000 B3PW91/def2-SVP_0003.out Cu 1.766528000000 0.077673000000 3.446894000000 Cu 1.838190000000 0.080910000000 -0.000045000000 Cu 0.077740000000 -1.766492000000 3.446913000000 Cu -0.077751000000 1.766506000000 -3.446912000000 Cu 1.763047000000 1.925365000000 -1.779435000000 Cu 1.925345000000 -1.763064000000 -1.779452000000 Cu 0.080910000000 -1.838190000000 -0.000032000000 Cu -1.766562000000 -0.077671000000 -3.446876000000 Cu 1.763093000000 1.925351000000 1.779389000000 Cu -1.925323000000 1.763058000000 1.779439000000 Cu 0.000037000000 -0.000025000000 1.682692000000 Cu -0.077625000000 1.766462000000 3.446919000000 Cu -1.965931000000 3.488041000000 0.000044000000 Cu -3.488044000000 -1.965952000000 0.000067000000 Cu -1.838188000000 -0.080910000000 0.000026000000 Cu -0.156811000000 3.562953000000 1.737296000000 Cu -0.156860000000 3.562950000000 -1.737260000000 Cu 3.562973000000 0.156828000000 1.737221000000 Cu -3.562936000000 -0.156827000000 1.737311000000 Cu 1.925390000000 -1.763066000000 1.779369000000 Cu -3.647044000000 1.651996000000 0.000059000000 Cu -0.080909000000 1.838182000000 0.000010000000 Cu 3.488038000000 1.965934000000 -0.000040000000 Cu 3.647048000000 -1.652008000000 -0.000063000000 Cu -3.562987000000 -0.156844000000 -1.737200000000 Cu -1.763100000000 -1.925366000000 -1.779384000000 Cu -1.766426000000 -0.077689000000 3.446934000000 Cu 0.156876000000 -3.562943000000 1.737251000000 Cu 1.766417000000 0.077706000000 -3.446935000000 Cu -1.925395000000 1.763077000000 -1.779374000000 Cu 0.077620000000 -1.766441000000 -3.446908000000 Cu 0.156808000000 -3.562953000000 -1.737295000000 Cu 1.652006000000 3.647036000000 -0.000003000000 Cu -0.000036000000 0.000014000000 -1.682706000000 Cu 1.965955000000 -3.488038000000 -0.000047000000 Cu -1.651997000000 -3.647052000000 0.000026000000 Cu 3.562941000000 0.156852000000 -1.737322000000 Cu -1.763036000000 -1.925362000000 1.779427000000 38 2.730879998 B3PW91/def2-SVP_0004.out Cu 1.882597000000 2.129958000000 1.676373000000 Cu -1.344741000000 3.519814000000 -0.719547000000 Cu 2.763553000000 2.763337000000 -0.756014000000 Cu 4.079672000000 0.704919000000 -1.669781000000 Cu -1.825838000000 0.692625000000 -0.133286000000 Cu 2.592148000000 -3.570855000000 -0.544308000000 Cu -0.514477000000 2.649208000000 1.801914000000 Cu -3.803023000000 -2.545118000000 -2.115802000000 Cu 2.542787000000 -2.963730000000 2.036260000000 Cu -3.269940000000 -1.516300000000 0.105120000000 Cu 0.105495000000 0.824799000000 3.446862000000 Cu 0.433737000000 -0.932992000000 -3.477843000000 Cu 1.713720000000 1.177590000000 -2.538706000000 Cu -1.934983000000 -3.076574000000 1.906265000000 Cu 0.397790000000 -2.776614000000 -1.602484000000 Cu 2.462087000000 -1.297824000000 -1.938485000000 Cu -3.832893000000 0.719471000000 1.127644000000 Cu -1.687445000000 -1.404913000000 -2.115556000000 Cu 3.873859000000 -1.305304000000 0.289817000000 Cu -3.621170000000 -0.035939000000 -1.832329000000 Cu -1.947140000000 -3.530526000000 -0.700198000000 Cu -2.315245000000 1.656362000000 3.147416000000 Cu 0.729291000000 3.543750000000 -2.205643000000 Cu -1.950057000000 -0.554628000000 2.243282000000 Cu -2.818606000000 2.919744000000 1.132268000000 Cu 4.189385000000 1.272501000000 0.880473000000 Cu -1.163673000000 1.352090000000 -2.401880000000 Cu 0.885195000000 4.184092000000 0.351302000000 Cu -3.371802000000 2.222983000000 -1.141821000000 Cu 2.401792000000 -0.331887000000 2.263422000000 Cu 0.278576000000 -3.765635000000 0.797566000000 Cu 0.264007000000 -1.789798000000 2.610879000000 Cu -0.910641000000 -1.679981000000 0.346427000000 Cu 2.306248000000 0.474655000000 -0.162050000000 Cu 0.404958000000 2.005649000000 -0.469152000000 Cu 0.251085000000 -0.291179000000 -1.233171000000 Cu 0.243686000000 0.279173000000 1.210476000000 Cu 1.510005000000 -1.722922000000 0.384287000000 38 2.919129997 B3PW91/def2-SVP_0005.out Cu -1.207309000000 -2.249324000000 -2.099540000000 Cu -2.553408000000 -0.079343000000 2.099096000000 Cu -1.987940000000 -3.704668000000 0.000010000000 Cu -1.207294000000 -2.249303000000 2.099529000000 Cu -0.135135000000 4.348504000000 1.260083000000 Cu 3.833218000000 -2.058011000000 1.259920000000 Cu -2.553434000000 -0.079342000000 -2.099107000000 Cu 3.698293000000 2.291305000000 1.259681000000 Cu 0.135134000000 -4.348507000000 -1.260086000000 Cu -0.135133000000 4.348510000000 -1.260087000000 Cu -4.202513000000 -0.130263000000 0.000017000000 Cu 1.343863000000 -2.168928000000 2.099842000000 Cu 0.000000000000 0.000000000000 1.337808000000 Cu 1.987939000000 3.704667000000 0.000010000000 Cu 4.202510000000 0.130260000000 0.000018000000 Cu 2.213837000000 -3.574171000000 0.000007000000 Cu -2.213836000000 3.574174000000 0.000007000000 Cu 2.553410000000 0.079340000000 2.099097000000 Cu 3.833248000000 -2.058021000000 -1.259889000000 Cu 1.207293000000 2.249304000000 2.099528000000 Cu 3.698331000000 2.291318000000 -1.259642000000 Cu -1.343874000000 2.168947000000 -2.099850000000 Cu -3.698296000000 -2.291308000000 1.259681000000 Cu 1.207308000000 2.249326000000 -2.099541000000 Cu -3.833247000000 2.058021000000 -1.259882000000 Cu 0.135137000000 -4.348501000000 1.260083000000 Cu -1.343860000000 2.168929000000 2.099840000000 Cu -3.698333000000 -2.291321000000 -1.259643000000 Cu -3.833218000000 2.058011000000 1.259915000000 Cu 1.343877000000 -2.168947000000 -2.099852000000 Cu 2.553434000000 0.079340000000 -2.099108000000 Cu 0.000000000000 0.000000000000 -1.337839000000 Cu -0.072798000000 2.344484000000 -0.000011000000 Cu -1.993429000000 -1.234903000000 -0.000023000000 Cu -2.066580000000 1.109229000000 -0.000019000000 Cu 1.993425000000 1.234900000000 -0.000023000000 Cu 0.072796000000 -2.344481000000 -0.000011000000 Cu 2.066580000000 -1.109230000000 -0.000020000000 38 2.946739998 B3PW91/def2-SVP_0006.out Cu 2.084683000000 0.677355000000 2.235209000000 Cu -4.238067000000 -0.055026000000 -1.102629000000 Cu 4.238067000000 -0.055026000000 -1.102629000000 Cu 0.000000000000 2.205357000000 -0.244668000000 Cu 1.296277000000 -1.784172000000 -0.244668000000 Cu -4.108780000000 1.335023000000 0.961854000000 Cu 1.257302000000 -4.047645000000 -1.102629000000 Cu 1.361968000000 4.013637000000 -1.102629000000 Cu 1.327722000000 1.827452000000 -2.355474000000 Cu 0.000000000000 -2.258855000000 -2.355474000000 Cu -2.084683000000 0.677355000000 2.235209000000 Cu -2.148299000000 -0.698025000000 -2.355474000000 Cu 2.148299000000 -0.698025000000 -2.355474000000 Cu -1.327722000000 1.827452000000 -2.355474000000 Cu 3.445437000000 -1.119490000000 1.005895000000 Cu 0.000000000000 -3.622747000000 1.005895000000 Cu -2.097420000000 0.681493000000 -0.244668000000 Cu 1.288405000000 -1.773338000000 2.235209000000 Cu -1.288405000000 -1.773338000000 2.235209000000 Cu 0.000000000000 4.320227000000 0.961854000000 Cu -1.257302000000 -4.047645000000 -1.102629000000 Cu 2.539365000000 -3.495137000000 0.961854000000 Cu 4.108780000000 1.335023000000 0.961854000000 Cu 0.000000000000 0.000000000000 3.471906000000 Cu -2.539365000000 -3.495137000000 0.961854000000 Cu 0.000000000000 0.000000000000 1.035123000000 Cu -1.361968000000 4.013637000000 -1.102629000000 Cu 2.129397000000 2.930864000000 1.005895000000 Cu -3.445437000000 -1.119490000000 1.005895000000 Cu 3.461012000000 -2.446556000000 -1.102629000000 Cu -3.461012000000 -2.446556000000 -1.102629000000 Cu 3.396325000000 2.535590000000 -1.102629000000 Cu -2.129397000000 2.930864000000 1.005895000000 Cu -3.396325000000 2.535590000000 -1.102629000000 Cu 2.097420000000 0.681493000000 -0.244668000000 Cu -1.296277000000 -1.784172000000 -0.244668000000 Cu 0.000000000000 2.191966000000 2.235209000000 Cu 0.000000000000 0.000000000000 -1.494815000000 38 3.288727499 B3PW91/def2-SVP_0007.out Cu -1.768641000000 0.005711000000 3.447266000000 Cu -1.839297000000 0.005986000000 0.000000000000 Cu 0.005711000000 1.768641000000 3.447266000000 Cu -0.005711000000 -1.768641000000 -3.447266000000 Cu -1.852436000000 -1.840850000000 -1.778936000000 Cu -1.840850000000 1.852436000000 -1.778936000000 Cu 0.005986000000 1.839297000000 0.000000000000 Cu 1.768641000000 -0.005711000000 -3.447266000000 Cu -1.852436000000 -1.840850000000 1.778936000000 Cu 1.840850000000 -1.852436000000 1.778936000000 Cu 0.000000000000 0.000000000000 1.683808000000 Cu -0.005711000000 -1.768641000000 3.447266000000 Cu 1.800221000000 -3.577993000000 0.000000000000 Cu 3.577993000000 1.800221000000 0.000000000000 Cu 1.839297000000 -0.005986000000 0.000000000000 Cu -0.011405000000 -3.563935000000 1.736603000000 Cu -0.011405000000 -3.563935000000 -1.736603000000 Cu -3.563935000000 0.011405000000 1.736603000000 Cu 3.563935000000 -0.011405000000 1.736603000000 Cu -1.840850000000 1.852436000000 1.778936000000 Cu 3.567682000000 -1.820760000000 0.000000000000 Cu -0.005986000000 -1.839297000000 0.000000000000 Cu -3.577993000000 -1.800221000000 0.000000000000 Cu -3.567682000000 1.820760000000 0.000000000000 Cu 3.563935000000 -0.011405000000 -1.736603000000 Cu 1.852436000000 1.840850000000 -1.778936000000 Cu 1.768641000000 -0.005711000000 3.447266000000 Cu 0.011405000000 3.563935000000 1.736603000000 Cu -1.768641000000 0.005711000000 -3.447266000000 Cu 1.840850000000 -1.852436000000 -1.778936000000 Cu 0.005711000000 1.768641000000 -3.447266000000 Cu 0.011405000000 3.563935000000 -1.736603000000 Cu -1.820760000000 -3.567682000000 0.000000000000 Cu 0.000000000000 0.000000000000 -1.683808000000 Cu -1.800221000000 3.577993000000 0.000000000000 Cu 1.820760000000 3.567682000000 0.000000000000 Cu -3.563935000000 0.011405000000 -1.736603000000 Cu 1.852436000000 1.840850000000 1.778936000000 38 3.328887499 B3PW91/def2-SVP_0008.out Cu 2.240200000000 1.224155000000 2.099540000000 Cu 0.060179000000 2.553931000000 -2.099096000000 Cu 3.689645000000 2.015686000000 0.000000000000 Cu 2.240200000000 1.224155000000 -2.099540000000 Cu -4.349396000000 0.102498000000 -1.260083000000 Cu 2.086720000000 -3.817666000000 -1.259920000000 Cu 0.060179000000 2.553931000000 2.099096000000 Cu -2.263487000000 -3.715384000000 -1.259681000000 Cu 4.349396000000 -0.102498000000 1.260083000000 Cu -4.349396000000 0.102498000000 1.260083000000 Cu 0.098721000000 4.203372000000 0.000000000000 Cu 2.178952000000 -1.327548000000 -2.099842000000 Cu 0.000000000000 0.000000000000 -1.337808000000 Cu -3.689645000000 -2.015686000000 0.000000000000 Cu -0.098721000000 -4.203372000000 0.000000000000 Cu 3.590684000000 -2.186952000000 0.000000000000 Cu -3.590684000000 2.186952000000 0.000000000000 Cu -0.060179000000 -2.553931000000 -2.099096000000 Cu 2.086720000000 -3.817666000000 1.259920000000 Cu -2.240200000000 -1.224155000000 -2.099540000000 Cu -2.263487000000 -3.715384000000 1.259681000000 Cu -2.178952000000 1.327548000000 2.099842000000 Cu 2.263487000000 3.715384000000 -1.259681000000 Cu -2.240200000000 -1.224155000000 2.099540000000 Cu -2.086720000000 3.817666000000 1.259920000000 Cu 4.349396000000 -0.102498000000 -1.260083000000 Cu -2.178952000000 1.327548000000 -2.099842000000 Cu 2.263487000000 3.715384000000 1.259681000000 Cu -2.086720000000 3.817666000000 -1.259920000000 Cu 2.178952000000 -1.327548000000 2.099842000000 Cu -0.060179000000 -2.553931000000 2.099096000000 Cu 0.000000000000 0.000000000000 1.337808000000 Cu -2.344964000000 0.055202000000 0.000000000000 Cu 1.219908000000 2.002640000000 0.000000000000 Cu -1.124707000000 2.058197000000 0.000000000000 Cu -1.219908000000 -2.002640000000 0.000000000000 Cu 2.344964000000 -0.055202000000 0.000000000000 Cu 1.124707000000 -2.058197000000 0.000000000000 38 3.719819999 B3PW91/def2-SVP_0009.out Cu -3.571762000000 1.809671000000 0.000000000000 Cu 0.000000000000 1.835970000000 0.000000000000 Cu -3.563116000000 0.000000000000 1.737394000000 Cu 3.563116000000 0.000000000000 -1.737394000000 Cu 1.845427000000 1.845427000000 -1.779671000000 Cu 1.845427000000 1.845427000000 1.779671000000 Cu 0.000000000000 0.000000000000 1.689627000000 Cu 3.571762000000 -1.809671000000 0.000000000000 Cu -1.845427000000 1.845427000000 -1.779671000000 Cu -1.845427000000 -1.845427000000 -1.779671000000 Cu -1.835970000000 0.000000000000 0.000000000000 Cu -3.563116000000 0.000000000000 -1.737394000000 Cu 0.000000000000 -1.769253000000 -3.449212000000 Cu 0.000000000000 -3.563116000000 1.737394000000 Cu 0.000000000000 -1.835970000000 0.000000000000 Cu -1.769253000000 0.000000000000 -3.449212000000 Cu 1.769253000000 0.000000000000 -3.449212000000 Cu -1.809671000000 3.571762000000 0.000000000000 Cu -1.809671000000 -3.571762000000 0.000000000000 Cu -1.845427000000 1.845427000000 1.779671000000 Cu 0.000000000000 -3.563116000000 -1.737394000000 Cu 0.000000000000 0.000000000000 -1.689627000000 Cu 0.000000000000 3.563116000000 -1.737394000000 Cu 0.000000000000 3.563116000000 1.737394000000 Cu 1.809671000000 -3.571762000000 0.000000000000 Cu 1.845427000000 -1.845427000000 1.779671000000 Cu -3.571762000000 -1.809671000000 0.000000000000 Cu -1.769253000000 0.000000000000 3.449212000000 Cu 3.571762000000 1.809671000000 0.000000000000 Cu 1.845427000000 -1.845427000000 -1.779671000000 Cu 3.563116000000 0.000000000000 1.737394000000 Cu 1.769253000000 0.000000000000 3.449212000000 Cu 0.000000000000 1.769253000000 -3.449212000000 Cu 1.835970000000 0.000000000000 0.000000000000 Cu 0.000000000000 1.769253000000 3.449212000000 Cu 0.000000000000 -1.769253000000 3.449212000000 Cu 1.809671000000 3.571762000000 0.000000000000 Cu -1.845427000000 -1.845427000000 1.779671000000 38 3.978977500 B3PW91/def2-SVP_0010.out Cu 0.000000000000 -2.552852000000 2.099540000000 Cu 2.212383000000 -1.277320000000 -2.099096000000 Cu 0.000000000000 -4.204339000000 0.000000000000 Cu 0.000000000000 -2.552852000000 -2.099540000000 Cu 2.175372000000 3.767692000000 -1.260083000000 Cu -4.350603000000 0.000082000000 -1.260083000000 Cu 2.212383000000 -1.277320000000 2.099096000000 Cu -2.175372000000 3.767692000000 -1.260083000000 Cu -2.175231000000 -3.767774000000 1.260083000000 Cu 2.175372000000 3.767692000000 1.260083000000 Cu 3.641231000000 -2.102266000000 0.000000000000 Cu -2.212383000000 -1.277320000000 -2.099096000000 Cu 0.000000000000 0.000000000000 -1.337808000000 Cu 0.000000000000 4.204531000000 0.000000000000 Cu -3.641065000000 2.102170000000 0.000000000000 Cu -3.641231000000 -2.102266000000 0.000000000000 Cu 3.641065000000 2.102170000000 0.000000000000 Cu -2.210835000000 1.276426000000 -2.099540000000 Cu -4.350603000000 0.000082000000 1.260083000000 Cu 0.000000000000 2.554640000000 -2.099096000000 Cu -2.175372000000 3.767692000000 1.260083000000 Cu 2.210835000000 1.276426000000 2.099540000000 Cu 2.175231000000 -3.767774000000 -1.260083000000 Cu 0.000000000000 2.554640000000 2.099096000000 Cu 4.350603000000 0.000082000000 1.260083000000 Cu -2.175231000000 -3.767774000000 -1.260083000000 Cu 2.210835000000 1.276426000000 -2.099540000000 Cu 2.175231000000 -3.767774000000 1.260083000000 Cu 4.350603000000 0.000082000000 -1.260083000000 Cu -2.212383000000 -1.277320000000 2.099096000000 Cu -2.210835000000 1.276426000000 2.099540000000 Cu 0.000000000000 0.000000000000 1.337808000000 Cu 1.172793000000 2.031369000000 0.000000000000 Cu 1.172821000000 -2.031354000000 0.000000000000 Cu 2.345614000000 -0.000016000000 0.000000000000 Cu -1.172793000000 2.031369000000 0.000000000000 Cu -1.172821000000 -2.031354000000 0.000000000000 Cu -2.345614000000 -0.000016000000 0.000000000000 38 4.384342500 B3PW91/def2-SVP_0011.out Cu 1.276478000000 -2.210925000000 2.099554000000 Cu 2.552956000000 0.000000000000 -2.099554000000 Cu 2.102153000000 -3.641035000000 0.000000000000 Cu 1.276478000000 -2.210925000000 -2.099554000000 Cu 0.000000000000 4.350864000000 -1.259862000000 Cu -3.767959000000 -2.175432000000 -1.259862000000 Cu 2.552956000000 0.000000000000 2.099554000000 Cu -3.767959000000 2.175432000000 -1.259862000000 Cu 0.000000000000 -4.350864000000 1.259862000000 Cu 0.000000000000 4.350864000000 1.259862000000 Cu 4.204305000000 0.000000000000 0.000000000000 Cu -1.276478000000 -2.210925000000 -2.099554000000 Cu 0.000000000000 0.000000000000 -1.337686000000 Cu -2.102153000000 3.641035000000 0.000000000000 Cu -4.204305000000 0.000000000000 0.000000000000 Cu -2.102153000000 -3.641035000000 0.000000000000 Cu 2.102153000000 3.641035000000 0.000000000000 Cu -2.552956000000 0.000000000000 -2.099554000000 Cu -3.767959000000 -2.175432000000 1.259862000000 Cu -1.276478000000 2.210925000000 -2.099554000000 Cu -3.767959000000 2.175432000000 1.259862000000 Cu 1.276478000000 2.210925000000 2.099554000000 Cu 3.767959000000 -2.175432000000 -1.259862000000 Cu -1.276478000000 2.210925000000 2.099554000000 Cu 3.767959000000 2.175432000000 1.259862000000 Cu 0.000000000000 -4.350864000000 -1.259862000000 Cu 1.276478000000 2.210925000000 -2.099554000000 Cu 3.767959000000 -2.175432000000 1.259862000000 Cu 3.767959000000 2.175432000000 -1.259862000000 Cu -1.276478000000 -2.210925000000 2.099554000000 Cu -2.552956000000 0.000000000000 2.099554000000 Cu 0.000000000000 0.000000000000 1.337686000000 Cu 0.000000000000 2.345454000000 0.000000000000 Cu 2.031223000000 -1.172727000000 0.000000000000 Cu 2.031223000000 1.172727000000 0.000000000000 Cu -2.031223000000 1.172727000000 0.000000000000 Cu 0.000000000000 -2.345454000000 0.000000000000 Cu -2.031223000000 -1.172727000000 0.000000000000 38 5.728447499 sapo_tripelte.out Cu 2.104971000000 1.810934000000 1.786347000000 Cu -0.920785000000 3.673153000000 -0.570957000000 Cu 3.101279000000 2.494120000000 -0.597229000000 Cu 4.187925000000 0.350995000000 -1.608592000000 Cu -1.740688000000 0.913318000000 -0.114805000000 Cu 2.214023000000 -3.720067000000 -0.761560000000 Cu -0.254788000000 2.596591000000 1.879457000000 Cu -4.147585000000 -1.963558000000 -2.099724000000 Cu 2.260582000000 -3.298281000000 1.829099000000 Cu -3.456123000000 -1.316446000000 0.182859000000 Cu 0.136508000000 0.571013000000 3.473494000000 Cu 0.262347000000 -0.640973000000 -3.504849000000 Cu 1.883496000000 1.127917000000 -2.468600000000 Cu -2.209859000000 -3.024099000000 1.757305000000 Cu 0.062092000000 -2.656015000000 -1.767559000000 Cu 2.307823000000 -1.396490000000 -2.021704000000 Cu -3.782753000000 1.004111000000 1.091212000000 Cu -1.920527000000 -0.982222000000 -2.101292000000 Cu 3.724253000000 -1.740556000000 0.215213000000 Cu -3.856323000000 0.392576000000 -1.483624000000 Cu -2.312115000000 -3.277106000000 -0.924262000000 Cu -2.168717000000 1.674171000000 3.115662000000 Cu 1.152456000000 3.548818000000 -2.033777000000 Cu -2.020892000000 -0.526310000000 2.207392000000 Cu -2.535307000000 3.086668000000 1.171644000000 Cu 4.336054000000 0.718018000000 1.004649000000 Cu -0.844051000000 1.681827000000 -2.342225000000 Cu 1.370527000000 4.041658000000 0.539962000000 Cu -3.068092000000 2.628460000000 -1.178321000000 Cu 2.356616000000 -0.689556000000 2.250456000000 Cu -0.073982000000 -3.819948000000 0.558015000000 Cu 0.087070000000 -1.975487000000 2.486229000000 Cu -1.097536000000 -1.612902000000 0.243229000000 Cu 2.382211000000 0.245098000000 -0.129372000000 Cu 0.684806000000 1.982695000000 -0.354252000000 Cu 0.218590000000 -0.227528000000 -1.228178000000 Cu 0.263881000000 0.189080000000 1.214062000000 Cu 1.312613000000 -1.863675000000 0.284592000000 38 9.873712501 B3PW91/def2-SVP_0012.out Cu 1.071797000000 1.039191000000 3.440671000000 Cu 1.670128000000 -0.195626000000 -1.292520000000 Cu 1.848556000000 2.272109000000 1.291536000000 Cu -0.149249000000 -1.011549000000 -4.215538000000 Cu -0.193359000000 -1.213779000000 0.000000000000 Cu 1.071797000000 1.039191000000 -3.440671000000 Cu -2.926697000000 1.291384000000 -2.100001000000 Cu -4.144992000000 -0.720394000000 -1.269998000000 Cu 1.670128000000 -0.195626000000 1.292520000000 Cu -0.793115000000 -0.054366000000 2.103497000000 Cu 0.137050000000 3.640344000000 0.000000000000 Cu 2.237839000000 -1.153156000000 3.426281000000 Cu -0.149249000000 -1.011549000000 4.215538000000 Cu -2.707087000000 -0.827681000000 3.360700000000 Cu -2.085961000000 -2.117034000000 1.295066000000 Cu 3.464683000000 0.842378000000 2.549647000000 Cu 1.953683000000 -2.401585000000 0.000000000000 Cu 3.464683000000 0.842378000000 -2.549647000000 Cu -0.618647000000 2.422829000000 2.090675000000 Cu 3.583831000000 0.908208000000 0.000000000000 Cu -0.373771000000 -3.539088000000 0.000000000000 Cu 0.393465000000 -2.314605000000 2.121537000000 Cu 3.823897000000 -1.259109000000 -1.260455000000 Cu 1.848556000000 2.272109000000 -1.291536000000 Cu -4.144992000000 -0.720394000000 1.269998000000 Cu -2.325514000000 0.108425000000 0.000000000000 Cu -1.385560000000 1.158710000000 4.120699000000 Cu -0.022367000000 1.227671000000 0.000000000000 Cu -1.385560000000 1.158710000000 -4.120699000000 Cu 0.393465000000 -2.314605000000 -2.121537000000 Cu -2.707087000000 -0.827681000000 -3.360700000000 Cu -0.793115000000 -0.054366000000 -2.103497000000 Cu 3.823897000000 -1.259109000000 1.260455000000 Cu -2.085961000000 -2.117034000000 -1.295066000000 Cu -0.618647000000 2.422829000000 -2.090675000000 Cu -2.926697000000 1.291384000000 2.100001000000 Cu 2.237839000000 -1.153156000000 -3.426281000000 Cu -2.157666000000 2.523643000000 0.000000000000 38 10.693227499 B3PW91/def2-SVP_0013.out Cu 0.675402000000 1.249212000000 -0.492475000000 Cu -2.464758000000 -4.137839000000 -0.060290000000 Cu -0.469092000000 1.310620000000 -2.773885000000 Cu 4.524120000000 -0.000617000000 0.955882000000 Cu -2.607112000000 0.000336000000 -2.964050000000 Cu -0.257136000000 4.145907000000 1.156285000000 Cu -2.463644000000 4.138473000000 -0.060401000000 Cu -1.429467000000 0.000153000000 -0.818879000000 Cu -1.313961000000 1.317350000000 3.172039000000 Cu 3.254164000000 -2.031884000000 1.767070000000 Cu 0.960178000000 -0.000105000000 3.449731000000 Cu -3.805944000000 -2.104611000000 0.640073000000 Cu 1.751484000000 -2.460017000000 -2.369341000000 Cu 4.026756000000 0.000043000000 -2.609294000000 Cu -3.805391000000 2.105661000000 0.640093000000 Cu -1.314228000000 -1.317023000000 3.172055000000 Cu -0.258169000000 -4.145841000000 1.156297000000 Cu 1.752356000000 2.459324000000 -2.369303000000 Cu 3.254608000000 2.030918000000 1.767370000000 Cu 0.806373000000 2.105143000000 2.073751000000 Cu -2.751648000000 0.000372000000 1.509650000000 Cu 0.805893000000 -2.105370000000 2.073744000000 Cu -0.365018000000 3.340808000000 -1.235886000000 Cu -1.432069000000 2.103715000000 0.772008000000 Cu 1.815697000000 -3.258006000000 0.089853000000 Cu -0.330760000000 0.000030000000 1.404384000000 Cu 3.156046000000 -1.256726000000 -0.702840000000 Cu -0.365992000000 -3.340762000000 -1.235855000000 Cu -1.432615000000 -2.103358000000 0.772047000000 Cu -3.810596000000 0.000538000000 -0.721979000000 Cu 0.675095000000 -1.249439000000 -0.492485000000 Cu 3.156350000000 1.256033000000 -0.702520000000 Cu -2.531905000000 2.103095000000 -1.527759000000 Cu -0.469486000000 -1.310553000000 -2.773893000000 Cu 2.119448000000 -0.000325000000 1.256405000000 Cu 1.661013000000 -0.000303000000 -2.479826000000 Cu -2.532504000000 -2.102456000000 -1.527743000000 Cu 1.816512000000 3.257501000000 0.089966000000 38 10.695110000 B3PW91/def2-SVP_0014.out Cu 0.493648000000 0.674054000000 1.249181000000 Cu 0.059891000000 -2.464496000000 -4.138138000000 Cu 2.773432000000 -0.469529000000 1.309926000000 Cu -0.953542000000 4.524129000000 0.000000000000 Cu 2.963496000000 -2.607632000000 0.000000000000 Cu -1.155857000000 -0.257213000000 4.146794000000 Cu 0.059891000000 -2.464496000000 4.138138000000 Cu 0.817181000000 -1.431538000000 0.000000000000 Cu -3.173134000000 -1.312900000000 1.316180000000 Cu -1.768682000000 3.253869000000 -2.029986000000 Cu -3.448350000000 0.963982000000 0.000000000000 Cu -0.639505000000 -3.805505000000 -2.104754000000 Cu 2.369677000000 1.751035000000 -2.460192000000 Cu 2.608289000000 4.028800000000 0.000000000000 Cu -0.639505000000 -3.805505000000 2.104754000000 Cu -3.173134000000 -1.312900000000 -1.316180000000 Cu -1.155857000000 -0.257213000000 -4.146794000000 Cu 2.369677000000 1.751035000000 2.460192000000 Cu -1.768682000000 3.253869000000 2.029986000000 Cu -2.073543000000 0.805649000000 2.105512000000 Cu -1.509933000000 -2.751284000000 0.000000000000 Cu -2.073543000000 0.805649000000 -2.105512000000 Cu 1.235758000000 -0.365563000000 3.341412000000 Cu -0.773220000000 -1.432162000000 2.104178000000 Cu -0.090414000000 1.816170000000 -3.256637000000 Cu -1.404641000000 -0.330448000000 0.000000000000 Cu 0.703553000000 3.155639000000 -1.256914000000 Cu 1.235758000000 -0.365563000000 -3.341412000000 Cu -0.773220000000 -1.432162000000 -2.104178000000 Cu 0.722224000000 -3.812153000000 0.000000000000 Cu 0.493648000000 0.674054000000 -1.249181000000 Cu 0.703553000000 3.155639000000 1.256914000000 Cu 1.527608000000 -2.531705000000 2.102999000000 Cu 2.773432000000 -0.469529000000 -1.309926000000 Cu -1.253641000000 2.119179000000 0.000000000000 Cu 2.480492000000 1.662278000000 0.000000000000 Cu 1.527608000000 -2.531705000000 -2.102999000000 Cu -0.090414000000 1.816170000000 3.256637000000