Spin-orbital liquid in Ba3CuSb2O9 stabilized by oxygen holes
Abstract
Both the Jahn-Teller distortion of Cu2+O6 octahedra and magnetic ordering are absent in hexagonal Ba3CuSb2O9 suggesting a Cu 3 spin-orbital liquid state. Here, by means of resonant x-ray scattering and absorption experiment, we show that oxygen 2 holes play crucial role in stabilizing this spin-orbital liquid state. These oxygen holes appear due to the “reaction” Sb5+Sb3+ two oxygen holes, with these holes being able to attach to Cu ions. The hexagonal phase with oxygen 2 holes exhibits also a novel charge-orbital dynamics which is absent in the orthorhombic phase of Ba3CuSb2O9 with Jahn-Teller distortion and Cu 3 orbital order. The present work opens up a new avenue towards spin-charge-orbital entangled liquid state in transition-metal oxides with small or negative charge transfer energy.
Mott insulators with =1/2 or =1/2 are expected to show a variety of quantum spin liquid states when magnetic orders are suppressed due to geometrical frustration and/or quantum fluctuation Kitaev2006 ; Lee2008 ; Balents2010 . Among them, RuCl3 with Ru3+(=1/2) hexagonal lattice exhibits a Kitaev spin liquid state under magnetic field due to Majorana quantization Do2017 ; Kasahara2018 ; Baskaran2007 ; Jackeli2009 ; Knolle2014 ; Nasu2015 . The Kitaev state can be realized by the strong spin-orbit interaction of 4 or 5 transition-metal ions on the hexagonal lattice Jackeli2009 . Another quantum spin liquid state on a hexagonal lattice is realized in Ba3CuSb2O9 with Cu2+(=1/2) Zhou2011 ; Nakatsuji2012 . The spin liquid phase in Ba3CuSb2O9 can be associated with fluctuation in the orbital sector Feiner1997 ; Li1998 ; Vernay2004 ; Nasu2008 in contrast to the Kitaev state where the orbital degeneracy is lifted due to the strong spin-orbit interaction.
Ligand holes, e.g. oxygen holes often present in oxides with, typically, high valence (or high oxidation state) of a metal (such as nominally Fe4+ in CaFeO3 CaFeO3 , Cu3+ in NaCuO2 Mizokawa1991 or Bi4+ in BaBiO3 BaBiO3_1 ; BaBiO3_2 ) lead to a lot of nontrivial effect, which largely determine the properties of corresponding solids Khomskii ; Green . Among them, novel types of magnetic ordering, like up-up-down-down structure of NiO3 ( - rare earths ions Mizokawa2000 ; spontaneous charge, or rather valence bond disproportionation in CaFeO3 CaFeO3 and BaBiO3 BaBiO3_1 ; BaBiO3_2 , and, last but not least, their apparently fundamental role in High-Tc superconductivity in cuprates (presumably connected with the formation of Zhang-Rice singlets ZhangRice ). Here we uncover yet another nontrivial effect due to the presence or oxygen holes: the suppression of cooperative Jahn-Teller (JT) distortion and magnetic ordering, typical for the strong JT ion Cu2+, with the resulting formation of spin-orbital liquid state in a very interesting material Ba3CuSb2O9 Nakatsuji2012 . We also show that, besides the suppression of the JT and magnetic ordering, oxygen holes lead to a very specific dynamic effects.
The octahedrally coordinated Cu2+ with electronic configuration is known as one of the classical JT active ions. Usually, divalent CuO6 octahedron in concentrated systems always leads to a cooperative orbital ordering with the concomitant lattice distortion (cooperative JT effect, see e.g. Ref. KugelKhomskii ). When the CuO6 octahedron is elongated along the -axis, the Cu 3 orbital with symmetry is destabilized and accommodates the Cu 3 hole. Ba3CuSb2O9 harbors orthorhombic phase Zhou2011 and hexagonal phase Nakatsuji2012 ; Katayama2015 . A very unusual and unexpected effect – the absence of JT distortion in a classical strong JT ion Cu2+ in octahedral coordination was discovered in the hexagonal Ba3CuSb2O9 by Nakatsuji et al Nakatsuji2012 . There are very convincing experimental indications of a dynamic character of the behaviour of Cu in this system Ishiguro2013 ; Han2015 . However, the real microscopic origin of this behaviour was not elucidated in these detailed studies. Just the usual dynamic JT effect can hardly explain this behaviour: it is usually realized in well-isolated JT centers, but never in concentrated systems. An extra puzzle is that the same material seems to exist in two different modifications. The orthorhombic phase behaves quite normally: it shows a cooperative JT distortion, no special dynamic effects, etc. On the other hand, the hexagonal phase has all these strange features discussed above. What is the microscopic reason for this very different behaviour, remains a puzzle. Here, we present new experimental data which shed light on these questions and which give us the possibility to solve all these puzzles. Notably, our spectroscopic investigations show that, whereas the orthorhombic phase contains the usual Cu2+ with all the conventional features thereof, in the hexagonal phase we see definite signatures of the presence of substantial oxygen hole character. Fluctuations, inevitably appearing due to motion of these oxygen holes hopping from site to site, suppress conventional long-range JT ordering and magnetic ordering and cause the dynamics seen in ESR and NMR Ishiguro2013 ; Han2015 .
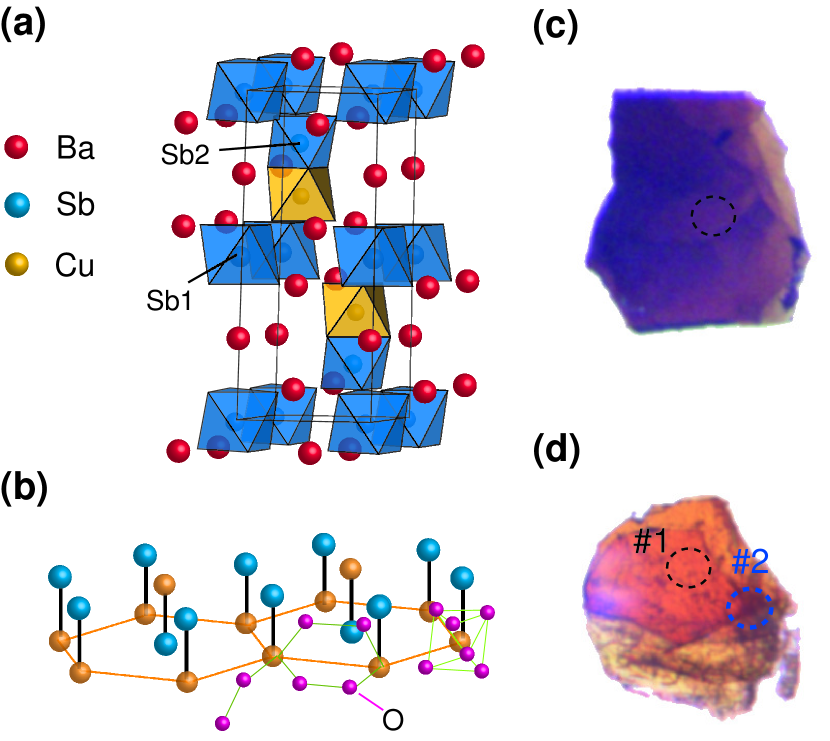
The basic crystal structure of Ba3CuSb2O9 is shown in Figs. 1(a) and 1(b) (hexagonal phase has symmetry ). Sb ions (blue balls/octahedra) occupy isolated octahedra and (in an ordered way) half of octahedra forming face-sharing dimers Nakatsuji2012 ; Katayama2015 . Cu ions (yellow) occupy other half of dimer octahedra, so that they form in effect the honeycomb lattice in the -plane as shown in Fig. 1(b). The structure of orthorhombic modification (symmetry ) is basically similar, but CuO6 octahedra are strongly distorted due to Jahn-Teller effect typical for Cu2+.
Single crystals of Ba3CuSb2O9 were grown under oxygen atmosphere from the BaCl2-based flux Katayama2015 . Two types of single crystals were obtained, depending on the growth condition. A small addition (9 mol %) of Ba(OH)2 to the BaCl2 flux was found to stabilize single-phase crystals of the hexagonal samples, whereas the pure BaCl2 flux leads to the growth of orthorhombic samples. The composition analysis by ICP-AES indicates that hexagonal samples are a single phase, and stoichiometric in terms of the Cu to Sb elemental ratio Katayama2015 . On the other hand, the orthorhombic crystals are rather offstoichiometric and seemed to contain a part of the inhomogeneous domain of the hexagonal phase, as discussed in the optical microscopy measurement. XAS and time-resolved soft x-ray scattering at the Cu (2 3) and O (1 2) absorption edges were conducted at BL07LSU in SPring-8 Takubo2017 . Further technical details about the optical and x-ray spectroscopies are described in the Supplementary Material supp .
An obvious difference between the hexagonal and orthorhombic phases is the ’color’ of the crystals. The crystal with dark color [Fig. 1(c)] is dominated by the hexagonal phase while the yellowish color crystal [Fig. 1(d)] mainly contains the orthorhombic phase. Figure 2(a) shows the optical absorption spectra for the hexagonal and orthorhombic crystals. The orthorhombic crystals exhibit inhomogeneity. The spectrum taken at position #1[see Fig. 1(d)] represents the orthorhombic phase with optical gap 2.0 eV which corresponds to the oxygen 2 to Cu 3 charge transfer excitation as expected in Cu2+ oxides. On the other hand, the spectrum at position #2 exhibits an intriguing absorption centered around 1.3 eV ( 950 nm) below the charge transfer excitation 2.0-3.0 eV. The appearance of the absorption peak in the charge transfer gap suggests that some holes are introduced in the Cu2+ Mott insulating state, similar to the high- cuprates. Surprisingly, in the absorption spectrum for the hexagonal phase, the in-gap absorption peak around 1.3 eV gains substantial spectral weight which causes the dark color. This indicates that a considerable number of holes are doped in the hexagonal phase although it is highly insulating.
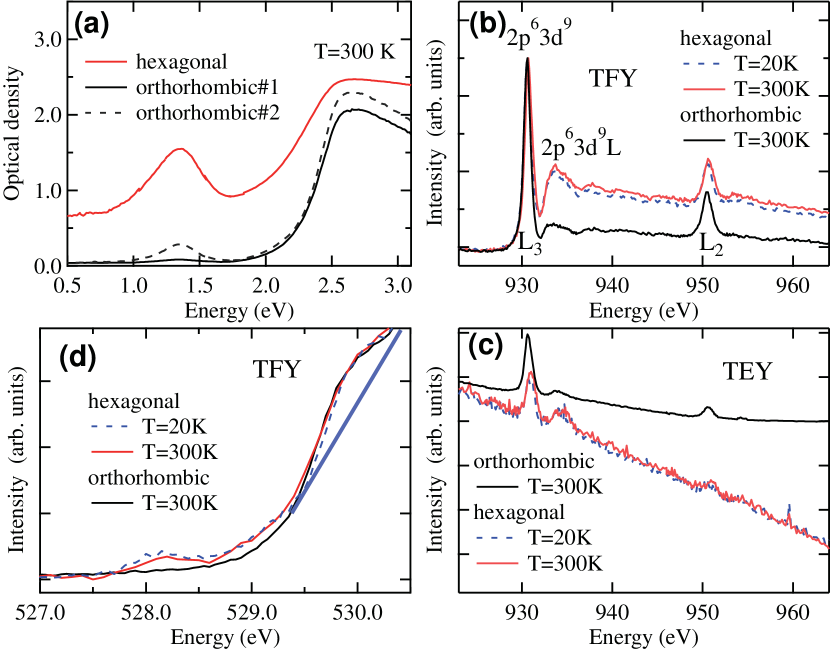
In order to further clarify this electronic structure difference between the hexagonal and orthorhombic phases, x-ray absorption spectroscopy have been investigated for both phases. Figure 2(b) shows the Cu 2 x-ray absorption spectra (XAS) in the total fluorescence yield mode. The Cu 2 main peak at 930.2 eV is accompanied by the charge transfer satellite at about 933 eV. The intensity of the satellite is enhanced in the hexagonal phase compared to the orthorhombic phase. The main and satellite peaks are assigned to the transitions of and of Mizokawa1991 . Here represents a hole on the oxygen 2 orbitals. The Cu 2 XAS indicates that the ground state of the hexagonal phase include more than the orthorhombic phase. Figure 2C shows the Cu 2 XAS taken in the total electron yield mode. The XAS spectra in the total electron yield mode are more surface sensitive than those in the total fluorescence yield mode. The XAS results obtained in the two modes are very consistent indicating that the contribution of is not due to the surface effect. In the hexagonal phase, the intensity of the satellite is comparable to that of the main peak indicting that the oxygen hole concentration is about 1/6 [see Fig. S3(a) in Supplementary Material]supp .
Figure 2(d) shows the oxygen 1 XAS spectra of the hexagonal and orthorhombic regions. The structure at 530 eV can be assigned to the transition from 1 to 2 of the oxygen which is hybridized into the upper Hubbard band (corresponding to ). The peak at 528.2 eV is observed only in the hexagonal region and can be assigned to the Zhang-Rice singlet band seen in the hole-doped high- cuprates: the transition from oxygen 1 to unoccupied oxygen 2 (corresponding to ) Chen1990 . The band around 1.3 eV seen in the optical absorption of Fig. 2(a) corresponds to the excitation from these state. The intensity of oxygen hole band (Zhang-Rice singlet band) in the O 1 XAS spectra is comparable to that of the upper Hubbard band after removing the Sb-O contribution which is roughly indicated by the blue line in Fig. 2(d). The comparison with the calculation suggests that the hole concentration per Cu site (or Sb2) site is around 1/6.
To get an extra information on the behaviour of our system, we carried out the time-resolved resonant x-ray scattering at 930.2 eV (on the Cu 2 to 3 resonance) after the pump pulse at 3.1 eV (Fig. 3). A coherent oscillation with period of 165 ps is clearly observed in the hexagonal phase, while no oscillation is seen in the orthorhombic phase. In addition, the x-ray scattering signals probed at 923.0 eV below the absorption edge do not show appreciable change after the pump pulse. The Fourier transform of the time-resolved data is plotted in Fig. 3(b) indicating that the coherent oscillation corresponds to 6 GHz which is rather slow compared to various optical phonons in the system which are usually coupled to charge or orbital orderings. Such a slow dynamics in the hexagonal phase is consistent with the previous reports Ishiguro2013 ; Han2015 .
The presence of the oxygen 2 hole in the hexagonal phase indicates that the Cu 3 spin and orbital are disturbed by hopping of the oxygen 2 holes. Since the hexagonal system with oxygen holes remains highly insulating, the oxygen hole should be confined within several Cu sites and disturbs the Cu 3 spin and orbital of those Cu sites. One of the possible units of confinement is the hexagonal cluster shown in Fig. 3(c) where the six CuO6 octahedra are connected through the Cu-O-O-Cu pathways. In this cluster, the oxygen hole and Cu 3 spins/orbitals form a quantum object keeping the hexagonal symmetry. As for the coherent oscillation seen in the time-resolved x-ray scattering in hexagonal samples, their source could be motion of oxygen holes in such clusters. The coherent oscillation with period of 165 ps is absent in the orthorhombic sample as shown in Fig. 3(b). Since the pump pulse with 3.1 eV corresponds to the charge transfer excitation from the O 2 to Cu 3 orbitals, the coherent oscillation is related to the charge dynamics in the Cu-O-O-Cu network. The most significant difference between the hexagonal and orthorhombic samples is the presence of oxygen hole in the hexagonal sample. Therefore, it is natural to assign the slow dynamics of 165 ps is derived from the oxygen hole in the Cu-O-O-Cu network.
There are several possible origins of the oxygen holes in Ba3CuSb2O9. The most plausible possibility is the high valence Sb in the Cu-Sb dimers. The formal valence is Cu2+ and Sb5+. However, the valence state 5+ is rather high for Sb. For such high oxidation states the real electronic configuration tends to contain ligand holes. For example, in BaBiO3 with Bi5+/Bi3+ mixed valence, it is established that the formally Bi5+ site has the actual electronic configuration close to Bi Foyevtsova2015 . The same trend is expected for Sb which is located above Bi in the periodic table. Indeed, the actual valence of Sb is rather close to 3+ as indicated by the HAXPES result presented in Supplementary Material supp . Therefore, the Sb 5 orbitals ’grab’ some electrons from the oxygen 2 orbitals and create oxygen 2 holes. If the oxygen 2 holes are bounded to the Sb site, the electronic configuration in the Cu-Sb dimer is essentially the same as Cu2+-Sb5+, i.e. we would have cluster Cu2+[Sb] with ligand holes forming something like Zhang-Rice state with Sb. However, this ligand hole can be also attached to Cu, making the state [Cu][Sb] or even [Cu]2Sb3+. In the simple schematic picture oxygen holes may be thus treated as moving in a double-well potential, one well centered on Cu and another, deeper well - on Sb, with the barrier in between. The average Sb-O bond length is about 2.004 Å for the Sb2 site while it is about 1.99 Å for the Sb1 site Katayama2015 . Consequently, the bond valence sum Palenik for the Sb1 and Sb2 sites are estimated to be 4.81 and 4.35, respectively. Therefore, the Sb2 site accommodates the extra electron to create the oxygen hole.
Fluctuation of ligand holes attached either to Sb or to Cu could be the reason of suppression of the long-range Jahn-Teller ordering of Cu in hexagonal phase. This is the main physics we deduce from our new experimental data, which, in our opinion, can explain the main features of the unexpected behavior of Ba3CuSb2O9. But even regardless of the origin of the oxygen hole, the present results rigorously prove that the oxygen hole and the Cu spins form the unique quantum object with spin-charge-orbital fluctuations of the specific time scale in the hexagonal phase. The Cu deficiency or the partial substitution of Cu by Sb was reported for the orthorhombic phase Katayama2015 . If one Cu2+ is replaced by Sb5+, three ligand holes can be eliminated. Therefore, the ligand holes are removed from the system due to the off-stoichiometry, and the Jahn-Teller distortion is recovered in the orthorhombic phase. This picture is indeed consistent with the O 1 XAS spectra in Fig. 2.
It is known that oxygen holes play significant roles in high valence transition-metal oxides such as Cu3+, Ni3+, and Fe4+ oxides. High valence transition-metal oxides with 90 degrees -O- bonds are insulating since the oxygen 2 hole mixed with the 3 hole in a O4 or O6 cluster cannot hop to neighboring clusters and is confined in the single-site cluster Mizokawa1991 . On the other hand, high valence transition-metal oxides with almost 180 degrees -O- bonds are metallic and their oxygen 2 holes are highly itinerant. With decreasing the -O- bond angle in NiO3 ( = rare earth metal) or FeO3 ( = alkaline earth metal) by reducing radius of the or ions, oxygen holes and transition-metal spins are ordered and form spin-charge ordered insulating states Mizokawa2000 ; Bisogni2016 . Compared to these other oxygen hole states, the oxygen hole state in Ba3CuSb2O9 is very unique in that due to a specific crystal structure with rather isolated CuO6 octahedra, the ligand holes have weaker tendency to become itinerant (even with large concentration of ligand holes the hexagonal samples of Ba3CuSb2O9 remain highly insulating). This can also lead to a significant difference of XAS spectra in this system as compared e.g. with the high- cuprates, see the discussion in the Supplementary Material supp .
In conclusion, x-ray and optical absorption experiments on Ba3CuSb2O9 reveal that the hexagonal phase with spin and orbital liquid is characterized by emergence of oxygen 2 holes in the highly insulating state. Their appearance originates due to tendency of Sb5+ to change electronic state by creating oxygen holes. Oxygen holes may fluctuate between Sb and Cu ions, or may be confined in the relatively large hexagonal cluster with six Cu sites, and the CuO6 JT distortion and long-range magnetic ordering are suppressed due to hopping of oxygen holes between Cu and Sb and between CuO6 octahedra. This can be a rather general situation in many materials containing different metal ions with relatively high valence, in which ligand (e.g. oxygen) holes can play crucial role in determining their properties. And the method we used - x-ray spectroscopy - can be one of the best methods to unravel interesting physics connected with these phenomena. Our results demonstrate strong interplay between electronic structure with the presence of oxygen holes in systems with unusual valencies, and the Jahn-Teller effect - the effect which often plays crucial role in concentrated solids including high- superconducting cuprates, but also in molecular systems and in inorganic chemistry. The coherent oscillations of 6 GHz, clearly observed in pump-probe resonant x-ray scattering, indicate that the spin and orbital fluctuations in the hexagonal phase have relatively slow dynamics, and suggests that the spin-charge-orbital fluctuation can be controlled by optical excitation. The present results also pave a new avenue towards optical control of the spin-charge-orbital states in transition-metal compounds with rich physical properties.
Acknowledgements
We are grateful for fruitful discussions with and support from S. Koshihara and T. Ishikawa. XAS and Tr-RSXS measurements were performed under the approval of Synchrotron Radiation Research Organization, the University of Tokyo (No. 2015A7401, 2018B7577). HAXPES measurements were performed under the approval of SPring-8 (Proposals No. Proposal No. 2018B1449). The work of D.Kh. is funded by the Deutsche Forschungsgemeinschaft (DFG, German Research Foundation) - Project number 277146847 - CRC 1238.
References
- (1) A. Kitaev, Anyons in an exactly solved model and beyond, Ann. Phys. 321, 2-111 (2006).
- (2) P. A. Lee, Physics. An end to the drought of quantum spin liquids, Science 321, 1306-1307 (2008).
- (3) L. Balents, Spin liquids in frustrated magnets, Nature 464, 199-208 (2010).
- (4) S.-H. Do, S.-Y. Park, J. Yoshitake, J. Nasu, Y. Motome, Y. S. Kwon, D. T. Adroja, D. J. Voneshen, K. Kim, T.-H. Jang, J.-H. Park, K.-Y. Choi, and S. Ji, Majorana fermions in the Kitaev quantum spin system -RuCl3, Nature Physics 13, 1079-1084 (2017).
- (5) Y. Kasahara, T. Ohnishi, Y. Mizukami, O. Tanaka, Sixiao Ma, K. Sugii, N. Kurita, H. Tanaka, J. Nasu, Y. Motome, T. Shibauchi, and Y. Matsuda, Majorana quantization and half-integer thermal quantum Hall effect in a Kitaev spin liquid, Nature 559, 227-231 (2018).
- (6) G. Baskaran, S. Mandal, and R. Shankar, Exact results for spin dynamics and fractionalization in the Kitaev model, Phys. Rev. Lett. 98, 247201 (2007).
- (7) G. Jackeli, and G. Khaliullin, Mott Insulators in the Strong Spin-Orbit Coupling Limit: From Heisenberg to a Quantum Compass and Kitaev Models, Phys. Rev. Lett. 102, 017205 (2009).
- (8) J. Knolle, D. L. Kovrizhin, J. T. Chalker, and R. Moessner, Dynamics of a two-dimensional quantum spin liquid: signatures of emergent Majorana fermions and fluxes, Phys. Rev. Lett. 112, 207203 (2014).
- (9) J. Nasu, M. Udagawa, and Y. Motome, Thermal fractionalization of quantum spins in a Kitaev model: temperature-linear specific heat and coherent transport of Majorana fermions, Phys. Rev. B 92, 115122 (2015).
- (10) H. D. Zhou, E. S. Choi, G. Li, L. Balicas, C. R. Wiebe, Y. Qiu, J. R. D. Copley, and J. S. Gardner, Spin liquid state in the S = 1/2 triangular lattice Ba3CuSb2O9, Phys. Rev. Lett. 106, 147204 (2011).
- (11) S. Nakatsuji, K. Kuga, K. Kimura, R. Satake, N. Katayama, E. Nishibori, H. Sawa, R. Ishii, M. Hagiwara, F. Bridges, T. U. Ito, W. Higemoto, Y. Karaki, M. Halim, A. A. Nugroho, J. A. Rodriguez-Rivera, M. A. Green, and C. Broholm, Spin-Orbital Short-Range Order on a Honeycomb-Based Lattice, Science 336, 559-563 (2012).
- (12) L. F. Feiner, A. M. Oles, and J. Zaanen, Quantum melting of magnetic order due to orbital fluctuations, Phys. Rev. Lett. 78, 2799 (1997).
- (13) Y. Li, Q. Ma, M. D. N. Shi, and F. C. Zhang, SU(4) theory for spin systems with orbital degeneracy, Phys. Rev. Lett. 81, 3527 (1998).
- (14) F. Vernay, K. Penc, P. Fazekas, and F. Mila, Orbital degeneracy as a source of frustration in LiNiO2, Phys. Rev. B 70, 014428 (2004).
- (15) J. Nasu, A. Nagano, M. Naka, and S. Ishihara, Doubly degenerate orbital system in honeycomb lattice: Implication of orbital state in layered iron oxide, Phys. Rev. B 78, 024416 (2008).
- (16) M. Takano, S. Nasu, T. Abe, K. Yamamoto, S. Endo, Y. Takeda, and J. B. Goodenough, Pressure-inuced high-spin to low-spin transition in CaFeO3, Phys. Rev. Lett. 67, 3267 (1991).
- (17) T. Mizokawa, H. Namatame, A. Fujimori, K. Akeyama, H. Kondoh, H. Kuroda, and N. Kosugi, Origin of the band gap in the negative charge-transfer-energy compound NaCuO2, Phys. Rev. Lett. 67, 1638 (1991).
- (18) T. M. Rice, and L. Sneddon, Real-Space and -Space Electron Pairing in BaPb1-xBixO3, Phys. Rev. Lett. 47, 689 (1981).
- (19) C. M. Varma, Missing valence states, diamagnetic insulators, and superconductors, Phys. Rev. Lett. 61, 2713 (1988).
- (20) D. I. Khomskii, Transition metal compounds. (Cambridge Univ.Press, 2014).
- (21) G. A. Sawatzky, and R. Green, ”The Explicit Role of Anion States in High-Valence Metal Oxides.” in Quantum Materials: Experiments and Theory Modeling and Simulation Vol. 6, E. Pavarini, E. Koch, J. van den Brink, and G. A. Sawatzky, Eds., (Verlag des Forschungszentrum, Julich, 2016) pp. 1–36.
- (22) T. Mizokawa, D. I. Khomskii, and G. A. Sawatzky, Spin and charge ordering in self-doped Mott insulators, Phys. Rev. B 61, 11263 (2000).
- (23) F. C. Zhang and T. M. Rice, Effective Hamiltonian for the superconducting Cu oxides, Phys. Rev. B 37, 3759(R) (1988).
- (24) K. I. Kugel’ and D. I. Khomskii, The Jahn-Teller effect and magnetism: transition metal compounds, Sov. Phys. Usp. 25, 231-256 (1982).
- (25) N. Katayama, K. Kimura, Y. Han, J. Nasu, N. Drichko, Y. Nakanishi, M. Halim, Y. Ishiguro, R. Satake, E. Nishibori, M. Yoshizawa, T. Nakano, Y. Nozue, Y. Wakabayashi, S. Ishihara, M. Hagiwara, H. Sawa, and S. Nakatsuji, Absence of Jahn-Teller transition in the hexagonal Ba3CuSb2O9 single crystal, Proc. Natl. Acad. Sci. U.S.A. 112, 9305-9309 (2015).
- (26) Y. Ishiguro, K. Kimura, S. Nakatsuji, S. Tsutsui, A. Q. R. Baron, T. Kimura, and Y. Wakabayashi, Dynamical spin-orbital correlation in the frustrated magnet Ba3CuSb2O9, Nature Comm. 4, 2022 (2013).
- (27) Y. Han, M. Hagiwara, T. Nakano, Y. Nozue, K. Kimura, M. Halim, and S. Nakatsuji, Observation of the orbital quantum dynamics in the spin-1/2 hexagonal antiferromagnet Ba3CuSb2O9, Phys. Rev. B 92, 180410(R) (2015).
- (28) K. Takubo, K. Yamamoto, Y. Hirata, Y. Yokoyama, Y. Kubota, S. Yamamoto, S. Yamamoto, I. Matsuda, S. Shin, T. Seki, K. Takanashi, H. Wadati, Capturing ultrafast magnetic dynamics by time-resolved soft x-ray magnetic circular dichroism, Appl. Phys. Lett. 110, 162401 (2017).
- (29) See Supplemental Material at http://link.aps.org/supplemental/ for additional experimental methods, data, and calculations.
- (30) C. T. Chen, F. Sette, Y. Ma, M. S. Hybertsen, E. B. Stechel, W. M. C. Foulkes, M. Schulter, S-W. Cheong, A. S. Cooper, L. W. Rupp, Jr., B. Batlogg, Y. L. Soo, Z. H. Ming, A. Krol, and Y. H. Kao, Electronic states in La2-xSrxCuO4+δ. probed by soft-x-ray absorption, Phys. Rev. Lett. 66, 104 (1991).
- (31) K. Foyevtsova, A. Khazraie, I. Elfimov, and G. A. Sawatzky, Hybridization effects and bond disproportionation in the bismuth perovskites, Phys. Rev. B 91, 121114 (2015).
- (32) V. Bisogni, S. Catalano, R. J. Green, M. Gibert, R. Scherwitzl, Y. Huang, V. N. Strocov, P. Zubko, S. Balandeh, J.-M. Triscone, G. Sawatzky, T. Schmitt, Ground-state oxygen holes and the metal-insulator transition in the negative charge-transfer rare-earth nickelates, Nature Commun. 7, 13017 (2016).
- (33) R.C. Palenik, K. A. Abboud and G. J. Palenik, Inorg. Chim. Acta 358, 1034 (2005).
- (34) NIST X-ray Photoelectron Spectroscopy Database, Version 4.1 (National Institute of Standards and Technology, Gaithersburg, 2012); http://srdata.nist.gov/xps/; DOI: http://dx.doi.org/10.18434/T4T88K.