New anomaly observed in 12C supports the existence and the vector character of the hypothetical X17 boson
Abstract
Employing the 11B(p,)12C nuclear reaction, the angular correlation of pairs was investigated in the angular range of for five different proton energies between Ep = 1.50 - 2.5 MeV. At small angles (), the results can be well interpreted by the internal pair creation process of electromagnetic radiations with E1 and M1 multipolarities and by the external pair creation in the target backing. However, at angles greater than , additional count excesses and anomalies were observed, which could be well accounted for by the existence of the previously suggested hypothetical X17 particle. Our results suggest that the X17 particle was generated mainly in E1 radiation. The derived mass of the particle is =17.03 MeV. According to the mass, and to the derived branching ratio (), this is likely the same X17 particle that we recently suggested for describing the anomaly observed in the decay of 8Be and 4He.
pacs:
23.20.Ra, 23.20.En, 14.70.PwI Introduction
Very challenging nuclear physics experiments were initiated in 1978 to detect a new particle, the axion, predicted by Weinberg we78 ; wi78 . The quantum chromodynamics axion is one of the most compelling solutions to the strong CP (charge conjugation parity symmetry) problem. Donelly do78 proposed to study the angular correlation of the pairs created in nuclear transitions as a signature for the decay of the axion. However, it was quickly ruled out in the MeV/c2 mass regime.
The later introduced dark photon ga84 ; ho86 is proposed as a force carrier connected to dark matter. In a minimal scenario, this new force can be introduced by extending the gauge group of the Standard Model with a new abelian U(1) gauge symmetry.
Pierre Fayet suggested a generalized dark photon model that would produce a light gauge boson with an extra U(1) gauge group already in 1980 Fayet:1980ad ; Fayet:1980rr ; Fayet:1980ss . Such a generalized dark photon may act as a mediator of light dark matter annihilations, possibly allowing for lighter than GeV/c2 dark matter particles Boehm:2003hm ; Fayet:2004bw . More recent discussions on the light U boson as the mediator of a new force, coupled to a combination of Q, B, L and dark matter can be found in Ref fa17 ; fa21 .
Searches for light particles, especially a 9 MeV/c2 particle suggested by Fokke de Boer and coworkers, have been performed in Frankfurt bo01 . These results, although with little confidence, appeared to confirm the existence of the 9 MeV/c2 particle. However, due to the closure of the accelerator, these experiments could not be continued.
Recently, we studied electron-positron angular correlations for the 17.6 MeV and 18.15 MeV transitions in 8Be and an anomalous angular correlation was observed for the 18.15 MeV transition kr16 . This was interpreted as the creation and decay of an intermediate bosonic particle with a mass of =16.700.35(stat)0.5(sys) MeV, which is now called X17. The possible relation of the X17 boson to the dark matter problem triggered an enormous interest in the wider physics community ins ; ag21 .
The first theoretical interpretation of the experimental results was performed by Feng et al. fe16 ; fe17 . They explained the anomaly with a 16.7 MeV, vector gauge boson X17, which may mediate a fifth fundamental force with some coupling to Standard Model (SM) particles. The theory of the dark photon has been generalized to the fact that the new particle is coupled not only to electric charges but also to quarks.
Constraints on the coupling constants of such a new particle, notably from searches for by the NA48/2 experiment ba15 was also taken into account by Feng and co-workers fe16 ; fe17 . Based on their results, the X17 particle couples much more strongly to neutrons than to protons, so the particle was named protophobic.
Zhang and Miller zh17 investigated the nuclear transition form factor as a possible origin of the anomaly, but they found the concluded form factor unrealistic for the 8Be nucleus.
Ellwanger and Moretti suggested another interpretation of the experimental results in view of a light, pseudoscalar particle ell16 . They predicted about ten times smaller branching ratio in case of the 17.6 MeV transition compared to the 18.15 MeV one, which is in nice agreement with our results.
Subsequently, many studies with different models have been performed including an extended two Higgs doublet model de17 ; de19 ; ro19 . They showed that the anomaly can be described with a very light Z0 bosonic state, with significant axial couplings.
In parallel to these recent theoretical studies, we re-investigated the 8Be anomaly with an improved experimental setup kra17 ; kra19 ; kr17 .
Recently, we also observed a similar anomaly in 4He kra19 ; kr21 . The signal could be described by the creation and subsequent decay of a light particle during the proton capture process on 3H to the ground state of the 4He nucleus. The derived mass of the particle ((stat.)(syst.) MeV) agreed well with that of the proposed X17 particle. It was also shown, that the branching ratios of the X17 particle compared to the -decay are identical within uncertainties for three beam energies, proving that the X17 particle was most likely formed in direct proton capture, which has a dominant multipolarity of E1. Our results obtained for 4He at different beam energies agree well with the present theoretical results of Viviani et al. vi21 .
Referring to our manuscript kr21 , Feng and co-workers have communicated a work very recently with the title of ,,Dynamical evidence for a fifth force explanation of the ATOMKI nuclear anomalies” fe20 , in which they propose to study the E1 ground state decay of the 17.2 MeV Jπ = 1- state in 12C in order to determine if X17 has a vector or axial-vector character.
In the present work, we investigated the 17.2 MeV transition of 12C to search for signatures of the creation of the X17 particle.
II Experimental methods
The experiments were performed in Debrecen, Hungary at the 2 MV Tandetron accelerator of ATOMKI. The Ex=17.23 MeV (Jπ = 1-) aj90 state of 12C was excited by the 11B(p,)12C nuclear reaction. Due to its large cross section, the reaction is also widely used for detector calibrations. The resonant proton energy is Ep = 1.388 MeV aj90 ; se65 .
Owing to the rather large level width ( = 1.15 MeV aj90 ), a 2 mg/cm2 thick 11B target was applied to maximize the yield of the pairs. The target was evaporated onto a 5 m thick Ta foil. The average energy loss of the protons in the target was 300 keV. To compensate for the energy losses, the energy of the protons was chosen to be Ep = 1.50, 1.70, 1.88, 2.10 and 2.50 MeV. The proton beam was impinged on a 11B target with a typical current of 2 A for about 50 hours at each beam energy. To achieve a more efficient cooling of the target and thus to reduce its degradation, we replaced the previously used kr16 ; gu16 Plexiglass support rods by Al rods. However, in turn, our data suffered a bit larger signal background from Al induced by the rays as shown in Fig. 1 than before (Fig. 9 of Ref. [17]).
Our previous detector setup kr16 ; gu16 has recently been upgraded. The details of the upgrade are described in kr21 . In the present experiment, the time and energy signals of the scintillators, as well as the time, energy and position signals of the DSSD detectors were recorded.
The energy calibration of the telescopes, the energy and position calibrations of the DSSD detectors, the Monte Carlo (MC) simulations as well as the acceptance calibration of the whole coincidence pair spectrometer were explained in Ref. kr21 . Reasonably good agreement was obtained between the experimental acceptance and results of the MC simulations, as presented in Fig. 1. The average difference is within % in the angular range of 40∘ - 170∘.
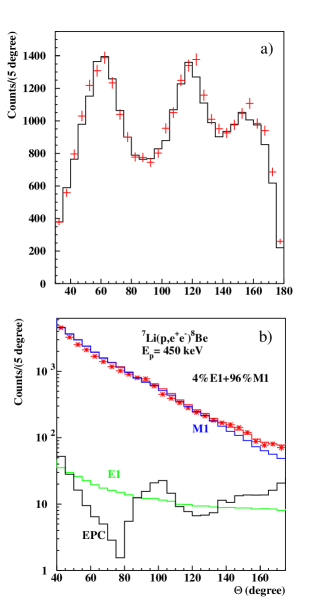
In order to validate the accuracy of the MC simulations, we performed measurements also on the 7Li(,)8Be reaction. The experimental results for the angular correlations from this data taking on the Ep = 441 keV resonance (red dots with error bars) are shown in Fig. 1 b), together with the corresponding Monte Carlo simulation (histogram) of the IPC process stemming mostly from the M1 nuclear transition. The contribution of the external pair creation (EPC) process of the 17.6 MeV -rays is also shown by a black histogram. We note here that the direct-capture contribution is negligible compared to the M1 IPC due to the large resonance capture cross section and the thin target.
As it can be seen in Fig. 1, the simulation of the IPC process manages to describe the shape of the data distribution accurately, and the contribution of EPC created on the different parts of the spectrometer is reasonably low.
In order to search for the assumed X17 particle, both the sum-energy spectrum of the pairs measured by the telescopes, and their angular correlations, determined by the DSSD detectors, have been analyzed. Since the counting rates in the detectors were low ( Hz in the scintillators and ( Hz in the DSSD detectors) and the coincidence time window was sharp ( ns) the effect of random coincidences was negligible. In the followings we show only the real coincidence gated spectra.
In the GEANT simulations, both pairs generated by internal pair creation in the target and the - pairs generated by external pair creation in the Ta backing were taken into account. A more detailed description of the simulations can be found in Ref. kr21 .
III Experimental results and discussion
The total energy spectrum of the -pairs produced in the decay of the Ex=17.2 MeV (Jπ = 1-) state of 12C at Ep=1.7 MeV is presented in Fig. 2. In addition to the E1 ground state transition, this state decays to the Ex=4.44 MeV (J) level with an E = 12.76 MeV E1 transition, which is also present the energy spectrum. The intense, 4.44 MeV ground state transition was discarded by setting a proper hardware threshold in order to reduce the high count rate of the DAQ.
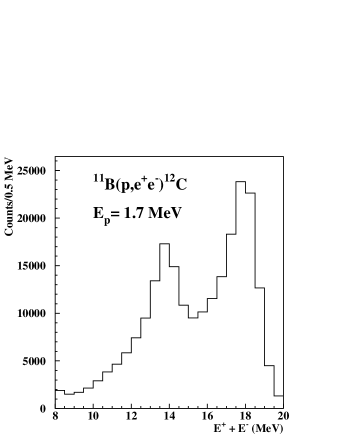
The experimental efficiency of the spectrometer was determined with uncorrelated -pairs by taking the and data from consecutive events as previously described in Refs. kr16 ; kr17 ; kra17 ; kr21 . The gated and efficiency-corrected angular correlation in the 17.2 MeV (J) transition is shown in Fig. 3 for proton energies of Ep= 1.5, 1.7, 1.88, 2.1 and 2.5 MeV.
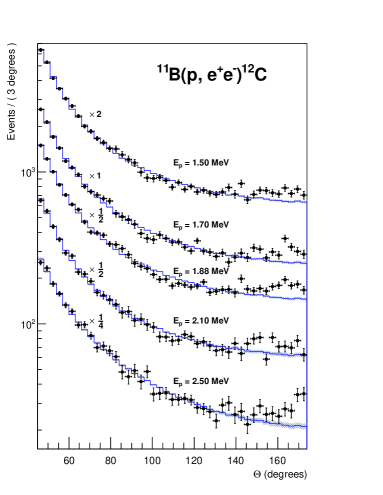
As show in Fig. 3, a combination of the MC simulated IPC distributions of E1 and M1 radiations together with a small contribution of simulated external pair creation (EPC) in the Ta backing can describe the experimental distributions below reasonable well. However, we observe significant deviations at large angles () at each proton energy.
To derive the invariant mass of the decaying particle, we carried out a fitting procedure for both the mass value and the amplitude of the observed peak.
The fit was performed with RooFit Verkerke:2003ir by describing the angular correlation with the following intensity function (INT):
(1) |
where was determined from a separate fit for the background region, was simulated by GEANT for the two-body decay of an X particle as a function of its mass, and and are the fitted numbers of background and signal events, respectively.
The signal PDF was constructed as a 2-dimensional model function of the opening angle and the mass of the simulated particle. To construct the mass dependence, the PDF linearly interpolates the opening angle distributions simulated for discrete particle masses.
Using the intensity function described in Equation 1, we first performed a list of fits by fixing the simulated particle mass in the signal PDF to a certain value, and letting RooFit estimate the best values for and . Allowing the particle mass to vary in the fit, the best fitted mass is calculated and the corresponding fit is shown for each studied beam energy in Fig. 4.
A significant background is obtained from the E1 transition, but the contribution from the assumed particle decay is also significant at large angles.
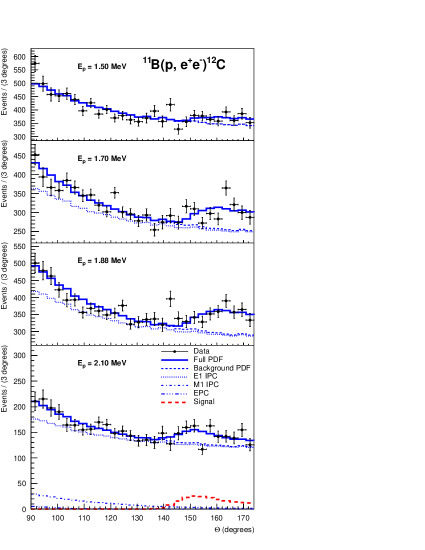
The measured invariant masses of the hypothetical X17 and the branching ratios (Bx) of its decay to the decay, as derived from the fits are summarized in Table 1. The values are compatible for each fitted parameter within 1 error bars. Their average values are also highlighted.
Ep | Bx | Mass | Confidence | |
---|---|---|---|---|
(MeV) | (MeV/c2) | |||
1.50 | 1.1(6) | 16.81(15) | 3 | |
1.70 | 3.3(7) | 16.93(8) | 7 | |
1.88 | 3.9(7) | 17.13(10) | 8 | |
2.10 | 4.9(21) | 17.06(10) | 3 | |
Averages | 3.6(3) | 17.03(11) | ||
Previous kr16 | 5.8 | 16.70(30) | ||
Previous kr21 | 5.1 | 16.94(12) | ||
Predicted fe20 | 3.0 |
In Table 1, only the statistical errors are indicated. The systematic uncertainties were estimated to be (syst.) MeV by employing a series of MC simulations as presented in one of our previous works kr21 . It mostly represents the uncertainty of the position of the beam spot, which was found to be shifted by about mm in one measurement run.
The extracted invariant mass agrees well with the values published earlier for the 8Be kr16 and the 4He kr21 experiments, which provides a convincing kinematic verification of the existence of the X17 particle. The branching ratio of the X17 decay differs from the previous data, but, on the other hand, agrees well with the theoretically predicted value fe20 .
IV Summary
We have studied the E1 ground state decay of the 17.2 MeV Jπ = 1- state in 12C. The energy-sum and the angular correlation of the pairs produced in the 11B(,e+e-)12C reaction were measured at proton energies of Ep= 1.50, 1.70, 1.88, 2.10 and 2.50 MeV. The gross features of the distributions of these quantities can be described well by the IPC process following the decay of the resonance and by considering a small contribution of external pair creation process induced by the high-energy rays. However, on top of the smooth, monotonic distribution of the angular correlation of pairs, we observed significant peak-like anomalous excess around 155-160∘ at four different beam energies.
The excess can be well-described by the creation and subsequent decay of the X17 particle, which we have recently suggested kr16 ; kr21 . The invariant mass of the particle was derived to be ((stat.)(syst.) MeV), which agrees well with our previously published values. The branching ratio of the decay of X17 to the decay was found to be . The present observation of the X17 particle in an E1 transition supports its vector character, as suggested by Feng et al. fe20 .
Given the present results on the X17 creation in E1 transitions, we consider to search for X17 in the decay of Giant Dipole Resonance (GDR) excitations of different nuclei.
V Acknowledgements
We wish to thank Z. Pintye for the mechanical and J. Molnar for the electronic design of the experiment. This work has been supported by the Hungarian NKFI Foundation No. K124810 and by the GINOP-2.3.3-15-2016-00034 and GINOP-2.3.3-15-2016-00005 grants.
References
- (1) S. Weinberg, Phys. Rev. Lett., 40, 223 (1978).
- (2) F. Wilczek, Phys. Rev. Lett., 40, 278 (1978).
- (3) T. W. Donnelly, S. J. Freedman, R. S. Lytel, R. D. Peccei, and M. Schwartz, Phys. Rev. D18, 1607 (1978).
- (4) P. Galison, A. Manohar, Phys. Lett. B 136 279 (1984).
- (5) B. Holdom, Phys. Lett. B. 166, 196 (1986).
- (6) P. Fayet, Phys. Lett. B 95 (1980), 285-289
- (7) P. Fayet, Nucl. Phys. B 187 (1981), 184-204
- (8) P. Fayet, Phys. Lett. B 96 (1980), 83-88
- (9) C. Boehm and P. Fayet, Nucl. Phys. B 683 (2004), 219-263
- (10) P. Fayet, Phys. Rev. D 70 (2004), 023514
- (11) Pierre Fayet, Eur. Phys. J. C 77 53 (2017).
- (12) Pierre Fayet, Phys. Rev. D 103, 035034 (2021).
- (13) F. W. N. de Boer et al., J. Phys. G27, L29 (2001).
- (14) A.J. Krasznahorkay et al., Phys. Rev. Lett. 116, 042501 (2016).
- (15) https://inspirehep.net/search?ln=en&p=refersto%3Arecid%3A1358248&jrec=26&sf=earliestdate
- (16) P. Agrawal et al., Eur. Phys. J. C 81, 1015 (2021).
- (17) J. L. Feng, B. Fornal, I. Galon, S. Gardner, J. Smolinsky, T.M.P. Tait, P. Tanedo, Phys. Rev. Lett. 117, 071803 (2016).
- (18) J.L. Feng, B. Fornal, I. Galon, S. Gardner, J. Smolinsky, T.M.P. Tait, P. Tanedo, Phys. Rev. D 95, 035017 (2017).
- (19) J. Batley et al., for the NA48/2 Collaboration, Phys. Lett. B 746, 178 (2015).
- (20) Xilin Zhang, Gerald A Miller Phys. Lett. B773 159 (2017).
- (21) U. Ellwanger and S. Moretti, JHEP 11 39 (2016).
- (22) Luigi Delle Rose et al., Phys. Rev. D 96, 115024 (2017) and references therein.
- (23) Luigi Delle Rose et al., Phys. Rev. D 99 055022 (2019).
- (24) Luigi Delle Rose et al., Frontiers in Physics 7 73 (2019).
- (25) A.J. Krasznahorkay et al., J. Phys.: Conf. Series 1056, 012028 (2017).
- (26) A.J. Krasznahorkay et al., Acta Phys. Pol. B 50, 675 (2019).
- (27) A.J. Krasznahorkay et al., Proceedings of Science, (Bormio2017) 036 (2017).
- (28) A.J. Krasznahorkay et al., Phys. Rev. C 104, 044003 (2021).
- (29) M. Viviani et al., Phys. Rev. C 105, 014001 (2022)
- (30) J.L. Feng, T.M.P. Tait and C.B. Verharen, Phys. Rev. D 102, 036016 (2020).
- (31) F Ajzenberg-Selove, Nucl. Phys. A506, 1 (1990).
- (32) R.E. Segel, S.S. Hanna, R.G. Allas, Phys. Rev. 139, B818 (1965).
- (33) J. Gulyás et al., Nucl. Instr. and Meth. in Phys. Res. A 808, 21 (2016).
- (34) W. Verkerke and D. P. Kirkby, “The RooFit toolkit for data modeling,” eConf C 0303241 (2003) MOLT007 [physics/0306116].