Force-free current sheets in the Jovian magnetodisk: the key role of electron field-aligned anisotropy
Abstract
Current sheets are an essential element of the planetary magnetotails, where strong plasma currents self-consistently support magnetic field gradients. The current sheet configuration is determined by plasma populations that contribute to the current density. The most commonly investigated configuration is supported by diamagnetic cross-field currents of hot ions, typical for the magnetospheres of magnetized planets. In this study, we examine a new type of the current sheet configuration supported by field-aligned currents from electron streams in the Jovian magnetodisk. Such bi-directional streams increase the electron thermal anisotropy close to the fire-hose instability threshold and lead to strong magnetic field shear. The current sheet configuration supported by electron streams is nearly force-free, with across the sheet. Using Juno plasma and magnetic field measurements, we investigate the internal structure of such current sheets and discuss possible mechanisms for their formation.
JGR: Space Physics
Department of Earth, Planetary, and Space Sciences, University of California, Los Angeles, USA Department of Atmospheric and Oceanic Sciences, University of California, Los Angeles, CA, USA Center for Space Physics, Boston University, Boston, MA, USA Southwest Research Institute, San Antonio, TX, USA Department of Physics and Astronomy, University of Texas at San Antonio, San Antonio, TX, USA Department of Physics, University of Texas at Dallas, Richardson, TX, USA
Anton [email protected]
We report Juno observations of thin anisotropic current sheets in the Jovian magnetodisk
The contribution of electron streams to the current sheet stress balance is estimated
We show force-free current sheet configuration supported by strong electron field-aligned currents
1 Introduction
Current sheets are observed in all planetary magnetotails, the night-side regions of stretched magnetic field lines. The configuration of magnetotails depends on characteristics of the planetary magnetic field interaction with solar wind [Bagenal (\APACyear1992), Bagenal \BBA Murdin (\APACyear2000), Jackman \BOthers. (\APACyear2014), Khurana \BBA Liu (\APACyear2018)], but all magnetotails contain current sheets, spatially localized regions of strong plasma currents. Instabilities of such current sheets, either internally or externally driven, can result in the magnetic reconnection that further transforms the magnetic energy to the plasma heating and charged particle acceleration [<]e.g.,¿Birn12:SSR,book:Gonzalez&Parker,Sitnov19. Among all the current sheets in space plasmas, the one in Earth’s magnetotail has been most intensively investigated, where strong diamagnetic currents, predominantly carried by hot protons (with a small fraction of oxygen ions), support the magnetic field configuration and pressure balance self-consistently (see Schematic in Fig. 1(a) and \citeABirn04,Sitnov&Merkin16,Zelenyi11PPR,ArtemyevZelenyi13,Lu16:CS). The current sheet in Earth’s magnetotail is characterized by large plasma ( is the ratio of plasma and magnetic field pressures), which leads to the dominant role of cross-field currents, , in the current sheet configuration. The relative contribution of ions and electrons to depends on the polarization electric fields, [Schindler \BBA Birn (\APACyear2002), Schindler \BOthers. (\APACyear2012)]; thin (ion-kinetic-scale) current sheets are, therefore, mostly electron dominated [Hesse \BOthers. (\APACyear1998), Runov \BOthers. (\APACyear2006), Artemyev \BOthers. (\APACyear2009), Lu \BOthers. (\APACyear2019)] due to the strong polarization electric field within [Zelenyi \BOthers. (\APACyear2010), Lu \BOthers. (\APACyear2016)]. However, in the Earth’s magnetotail, there are two interesting exceptions that can be appreciably large. First, in the near-Earth magnetotail, during the current sheet thinning (formation of thin current sheets during the substrom growth phase, see \citeABirn04MHD,Petrukovich07,Hsieh&Otto15) the spatial scale (thickness) of the current sheet can become smaller than the thermal proton gyroradius. In such sub-ion scale thin current sheets the proton pressure cannot be redistributed within sub-gyroradius scale. To establish the pressure balance, the intensified field-aligned electron currents form strong magnetic field shear, contributing to a (partially) force-free current sheet configuration with (see Schematic in Fig. 1(b) and \citeANakamura08,Artemyev13:jgr,Artemyev17:grl:currents). Second, a similar, partially force-free current sheet configuration has been observed in the distant (lunar orbit) magnetotail [Xu \BOthers. (\APACyear2018)] where plasma can be as low as , and plasma pressure is not sufficient to establish the pressure balance. This second force-free current sheet with low is also typical in Mars [Artemyev, Angelopoulos, Halekas\BCBL \BOthers. (\APACyear2017), DiBraccio \BOthers. (\APACyear2015)] and Venus [Rong \BOthers. (\APACyear2015)] magnetotails occupied by cold planetary plasmas.
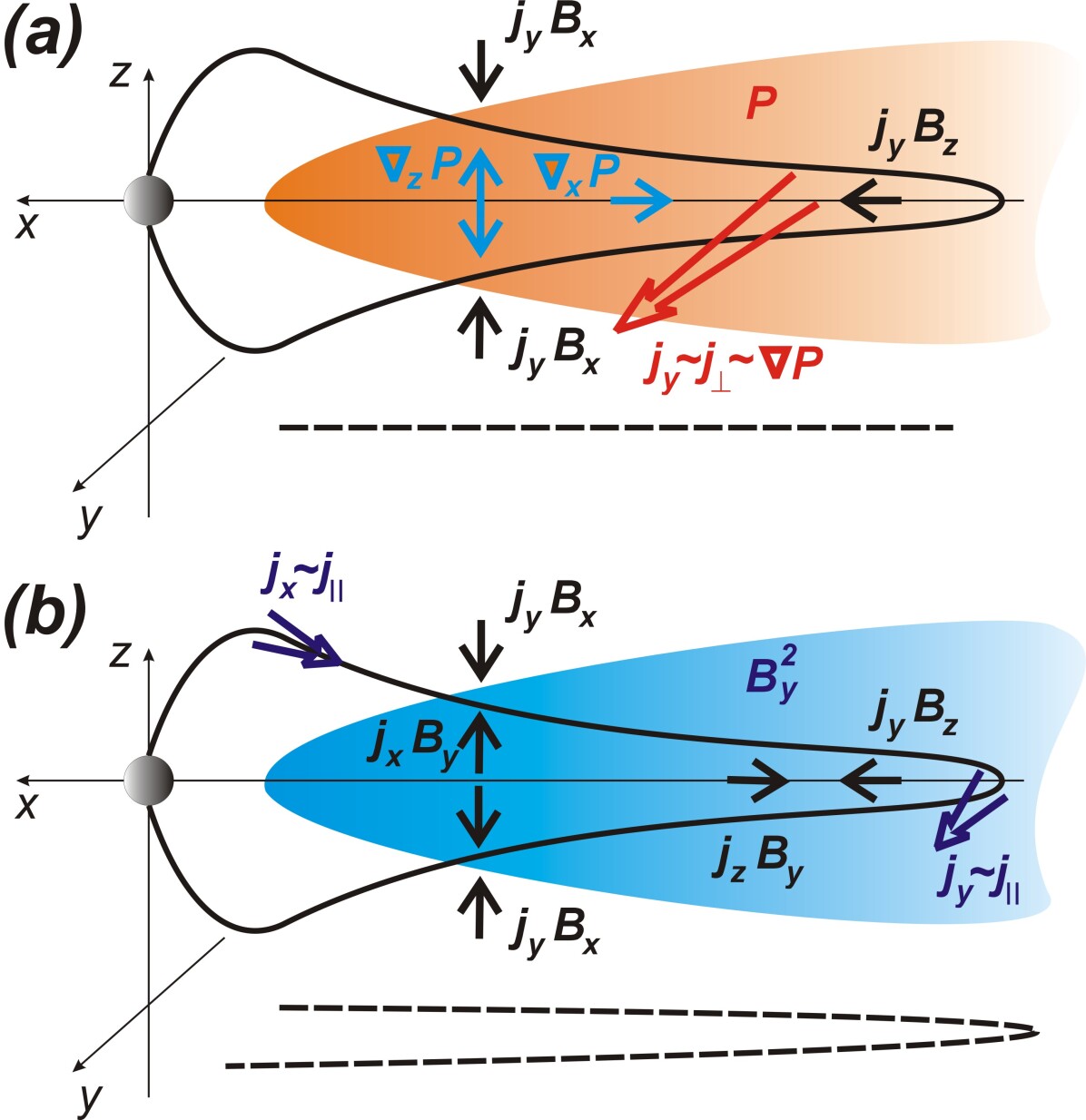
Between the two mechanisms for the force-free (or partially force-free) current sheet formation, sub-ion scale thickness versus low plasma , the first one is more interesting, because such sub-ion current sheets with strong field-aligned electron currents can be favorable to kinetically-driven magnetic field reconnection and current filamentation [Drake \BBA Lee (\APACyear1977), Zelenyi \BBA Taktakishvili (\APACyear1987), Wilson \BOthers. (\APACyear2016)]. Investigations of such current sheet configurations, however, are quite limited, because these current sheets are rather transient (dynamical) in the Earth’s magnetotail [<]see discussions in¿Nakamura08. An alternative plasma environment to investigate these specific current sheets would require hot heavy ions, curved magnetic field lines, and fast electron plasma flows. The best possible, accessible system is the Jovian magnetodisk, filled by sulfur and oxygen ions [Thomas \BOthers. (\APACyear2004), Krupp \BOthers. (\APACyear2004), Mauk \BOthers. (\APACyear2004), Kim \BOthers. (\APACyear2020\APACexlab\BCnt1)] with various charge states [<]e.g.,¿Selesnick09, Clark16, Allen19,Kim20:juno:PS and conjugate to the Jovian aroural region, a powerful source of field-aligned electron streams [<]e.g.,¿Mauk17,Mauk17:nature. Therefore, We will use the recently available plasma and magnetic field measurements [Bagenal \BOthers. (\APACyear2017)] from Juno in the Jovian magnetodisk to systematically examine sub-ion scale, force-free (or partially force-free) current sheets.
In this study, we focus on 18 events of Juno current sheet crossings during the first 30 orbits, i.e., those with a strong magnetic shear (field-aligned currents), electron field-aligned streams, and different combinations of proton and heavy ion contributions to the pressure. We estimate the current sheet spatial scale (thickness) and current density during its flapping motion. The following of paper includes three sections: description of Juno instruments and data analysis techniques in Sect. 2, detailed analysis of 9 current sheet crossings in Sect. 3, and discussion on the results in Sect. 4.
2 Data analysis technique and instruments
We use data from the Juno magnetometer (MAG) in 2017-2018, with s time resolution [Connerney, Benn\BCBL \BOthers. (\APACyear2017), Connerney, Adriani\BCBL \BOthers. (\APACyear2017)]. We focus on measurements at radial distances in the Jovian magnetodisk. Figure 2 shows a typical one-day magnetic field measurements in the magnetodisk by Juno: quasi-periodic crossings of zeros of the radial magnetic field component (current sheet) are due to flapping oscillations of the magnetodisk. For each such crossing we transform the magnetic field into local coordinate systems [Sonnerup \BBA Cahill (\APACyear1968)]: is the most varying magnetic field component, is the less varying component, and is transverse to and . We keep only those current sheets with a peak at and with available plasma measurements by the Jovian Auroral Distributions Experiment (JADE) instrument. The times of selected crossings are given in table 1, along with their radial distances. Overview plots of plasma and magnetic field profiles during each current sheet crossing are provided in the Supporting Information. In the main text, we mostly discuss six force-free current sheets, in comparison with three current sheets supported by plasma pressure gradients (non-force-free sheets), but our conclusions are supported by the entire dataset.
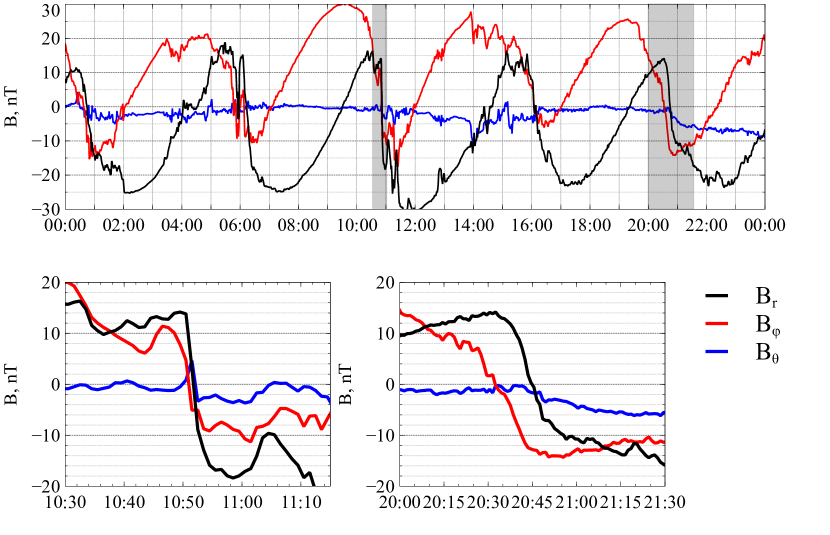
Jovian Auroral Distributions Experiment (JADE) measures [<]see¿McComas17:ssr,McComas17,Kim20:juno,Kim20:juno:PS electron distributions from below to keV (at a s cadence) and ions from eV to keV (including ion composition, at a s cadence). We have averaged JADE data over the spin period ( s) to obtain a complete pitch angle coverage from to . These energy ranges cover the main (thermal) plasma populations in Jupiter’s magnetodisk. We use the following data products from JADE: electron pitch-angle/energy distributions averaged over time interval of the current sheet crossing, electron omnidirectional energy spectra (energy flux) , electron pressure and density profiles, electron pressure anisotropy averaged over the current sheet crossing interval, omnidirectional proton and heavy ion energy spectrum (energy flux) and , and proton and heavy ion densities , . Note that field view of the JADE electron detector may not cover the entire pitch-angle range at the current sheet boundaries, so we average electron measurements over each current sheet crossing interval to obtain a more complete pitch-angle distribution. The rotation of the background magnetic field direction across the current sheet ensures a wide coverage of the pitch-angle range (see pitch-angle distributions below), which is needed for the estimate of . In this study, we use the heavy ion (or ”i”) to denote integrated quantities of those ion populations with mass-to-charge ratio larger than five. We also estimate proton and heavy ion pressures , as moments of the omnidirectional energy spectrum, i.e., we assume that thermal proton and ion speeds well exceed their bulk flow speed [<]this is a reasonable assumption, see¿Kane99,Frank02Jupiter,Waldrop05,Kim20:juno:PS.
# | date | time | radial | comments |
---|---|---|---|---|
distance | ||||
1 | 2017 doy 027 | 09:00-09:20 | CS, heavy ions | |
2 | 2017 doy 080 | 07:10-07:40 | CS, heavy ions | |
3 | 2017 doy 080 | 17:10-17:50 | non- CS, heavy ions | |
4 | 2017 doy 128 | 08:00-08:30 | CS, heavy ions | |
5 | 2017 doy 128 | 16:30-17:30 | CS, heavy ions | |
6 | 2017 doy 133 | 05:10-06:10 | non- CS, heavy ions | |
7 | 2017 doy 181 | 06:50-07:20 | CS, heavy ions | |
8 | 2017 doy 181 | 09:45-10:20 | CS, heavy ions | |
9 | 2018 doy 031 | 09:30-10:40 | non- CS, heavy ions | |
10 | 2018 doy 034 | 13:30-14:30 | non- CS, heavy ions | |
11 | 2018 doy 085 | 21:00-21:50 | non- CS, protons | |
12 | 2018 doy 086 | 10:15-11:15 | CS, heavy ions & protons | |
13 | 2018 doy 088 | 01:20-02:30 | CS, protons | |
14 | 2018 doy 088 | 11:30-12:30 | CS, protons | |
15 | 2018 doy 088 | 21:30-22:30 | CS, heavy ions | |
16 | 2018 doy 141 | 10:30-11:15 | CS, heavy ions & protons | |
17 | 2018 doy 141 | 19:45-21:00 | CS, heavy ions & protons | |
18 | 2018 doy 142 | 20:00-20:45 | non- CS, heavy ions & protons |
Using plasma and magnetic field measurements, we estimate profiles and electron fire-hose parameter . To show that this parameter controls the contribution of the electron anisotropy to the current density, we illustrate the case for a simple quasi-1D current sheet with , , and . The electron current due to cross-field drifts in such current sheet is [Shkarofsky \BOthers. (\APACyear1966)]
(1) |
where is the transverse component of the polarization electric field. This equation should be supplemented by the field-aligned stress balance equation
where is the field-aligned component of the polarization electric field and .
For force-free current sheets with , the current density equation can be rewritten as
whereas the parallel current density can be obtained from the divergence free condition:
Thus, the total electron current is
where .
3 Current sheet examples
Figure 3 shows six typical examples of thin current sheets with an almost constant magnetic field magnitude across the sheet, . Such a constant magnetic field implies the dominant role of field-aligned currents in the current sheet configuration: if , then and there is no pressure variation across the sheet [<]note that typical crossings of the magnetodisk current sheet show strong variations of the plasma pressure (density) across the sheet, see, e.g.,¿Bagenal21:Huscher_plasmasheet. Panels (a) show that is due to peak of component that compensates the drop of around the neutral plane (where ). As expected for the force-free current sheet, there are no appreciable variations in the ion fluxes (protons or heavy ions) across the sheet (see panel (c)). Electron fluxes may show some variations (see panel (b)), but variations of the electron thermal pressure are insufficient to cause any significant variations of the magnetic field pressure (see panel (d); note that a variation of corresponds to nT variation of the magnetic field).
Let us explain the absence of ion pressure variations during the observed current sheet crossings. As shown in Fig. 3, the time-scale of current sheet crossings varies from min to min; taking into account the flapping speed of , this time-scale can be converted to a spatial scale (here is the Jupiter rotational frequency, is the radial distance of the current sheet, and is the tilt angle of the magnetodisk with respect to the planetary equator, see \citeAConnerney98,Khurana05). Despite that we used the upper limit for the flapping speed [<]see discussion and observations in¿Hill79,Kim20:juno:PS, this spatial scale is much smaller than the typical thicknesses of Jovian magnetodisk current sheets, km [Connerney \BOthers. (\APACyear2020), Liu \BOthers. (\APACyear2021), Khurana \BOthers. (\APACyear2022)]. More importantly, this spatial scale is comparable to (or smaller than) the hot proton or heavy ion gyroradius: for the equatorial field of a typical current sheet, nT, keV protons and sulfur ions have gyroradii of km and km, respectively. Thus, these current sheets are likely on sub-ion scale, within which ions cannot redistribute their pressure. To establish the pressure balance in such current sheets, the field-aligned electron currents create a local peak.
To estimate the electron contribution to the field-aligned currents, we use Eq. (LABEL:eq:currents_par) and the measured electron pitch-angle, energy distributions. Figure 4(b) shows that all six current sheets are characterized by field-aligned bi-directional electron streams. These streams occupy in pitch angles around the parallel and anti-parallel (with respect to the background magnetic field) directions, in the energy range below keV. Such field-aligned streams may be generated by reconnection further downtail [<]e.g.,¿Kronberg12:icarus or originate from the aurora acceleration region [Mauk \BOthers. (\APACyear2017\APACexlab\BCnt2), Mauk \BOthers. (\APACyear2020), Elliott \BOthers. (\APACyear2020), Allegrini \BOthers. (\APACyear2020)]. In the Earth’s magnetosphere, similar field-aligned streams are observed in the near-Earth magnetotail [Hada \BOthers. (\APACyear1981), Walsh \BOthers. (\APACyear2013), Artemyev, Angelopoulos, Liu\BCBL \BBA Runov (\APACyear2017)], but their energies are well below keV, in agreement with the capability of the Earth’s aurora acceleration [Ergun \BOthers. (\APACyear2004), Chaston \BOthers. (\APACyear2007), Watt \BBA Rankin (\APACyear2009)]. More effective aurora acceleration in the Jupiter magnetosphere may produce keV beams [Kollmann \BOthers. (\APACyear2018), Saur \BOthers. (\APACyear2018), Damiano \BOthers. (\APACyear2019), Lysak \BOthers. (\APACyear2021)], which are likely further expanded in the pitch-angle space by various scattering mechanisms and form the electron streams observed in the plasma sheet [<]see discussion in¿Zhang20:jupiter. In the presence of a large electron , such field-aligned streams create a strong pressure anisotropy with the fire-hose parameter reaching one (see Fig. 4(c)). Thus, Eq. (LABEL:eq:currents_par) shows that for this large , electrons will carry almost all the current to support and variations across the sheet.
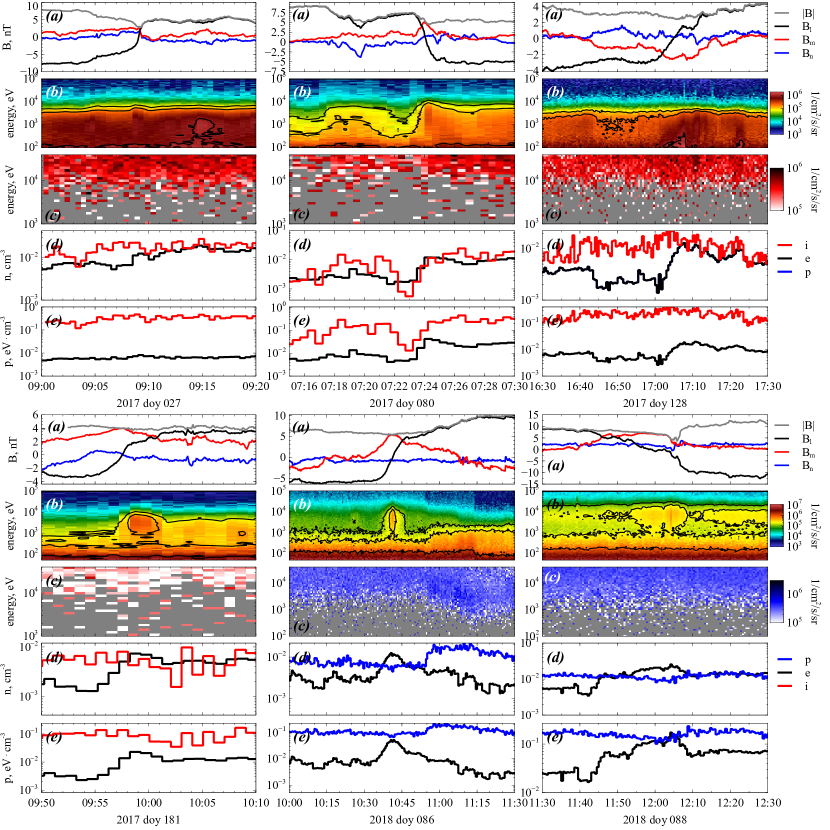
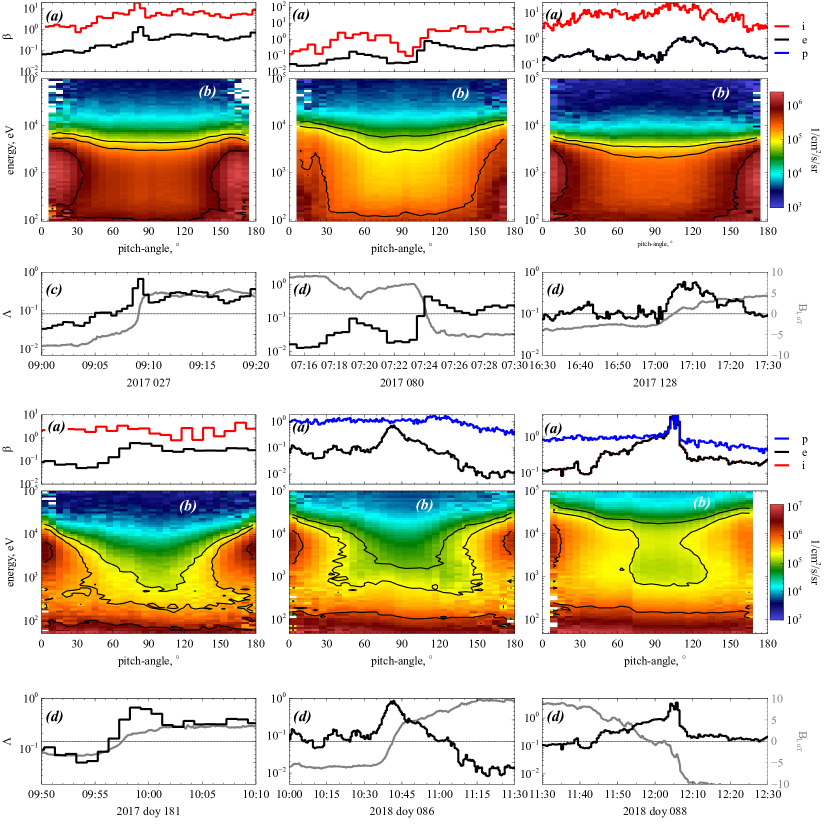
We explain the formation of force-free (partial force-free) current sheets with strong field-aligned electron currents (shown in Fig. 4) as a need to balance the magnetic field pressure decrease on a sub-ion scale. This explanation implies that similar electron currents should be observed in current sheets on larger scales, where they will not create peaks, but rather contribute to the cross-field current density, in agreement with Eq. (1) [<]see discussion in¿Artemyev16:pop:cs. Figure 5 shows such current sheets with a significant ion pressure contribution to the stress balance and strong field-aligned electron anisotropy. There is almost no peak in the current sheet center (where , see panel (a)), whereas ion fluxes and pressures exhibit peaks (panels (c,d)). Temporal scales of current sheet crossings from Fig. 5 are about 20-30min, corresponding to a spatial scale larger than the typical proton and heavy ion gyroradius in these current sheets. Note the two current sheets on 2017 doy 080 (shown in Fig. 3 and 5) exhibit different characteristics: the force-free current sheet in Fig. 3 was crossed within a couple of minutes and shows no variations, whereas the one in Fig. 5 was crossed within min and shows strong variations.
In current sheets from Fig. 5, electron pitch-angle distributions contain strong field-aligned streams with characteristics very similar to those in the force-free current sheets (compare Figs. 4(b) and 5(g)). However, contrary to force-free current sheets, these field-aligned streams mostly contribute to anisotropic cross-field electron currents, see Eq. (1). Indeed, small magnetic field pressure at the current sheet center (where ) increases and leads to a large electron fire-hose parameter even for a moderate anisotropy (see Fig. 5(f,h)).
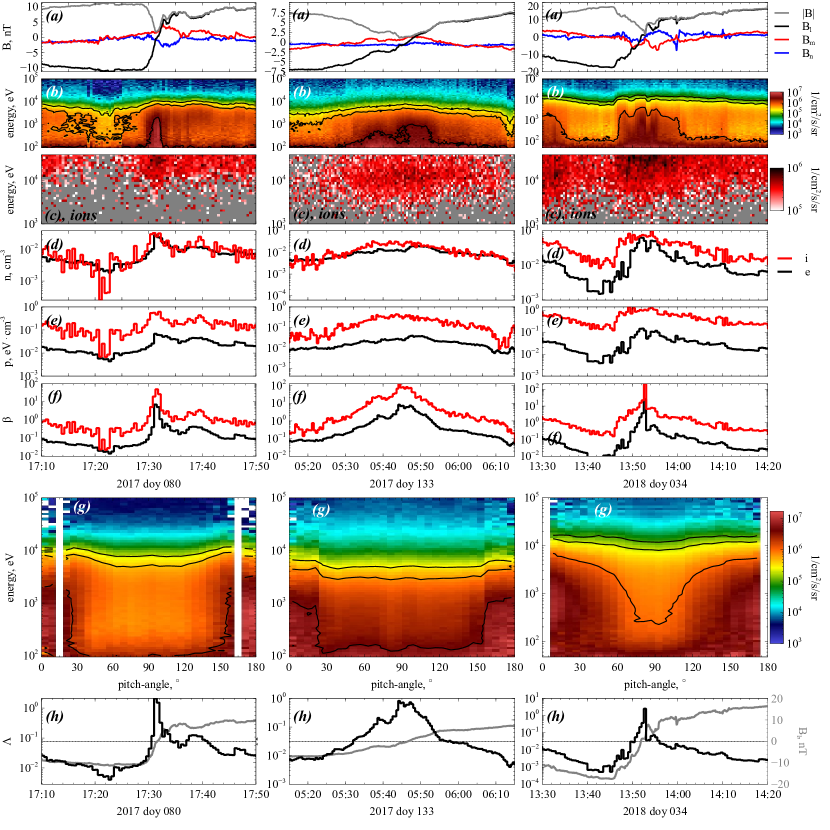
Comparison of Figs. 3, 4, and 5 suggests the following mechanism for the formation of the force-free current sheet. Certain external conditions (e.g., electron acceleration in the aurora region, see \citeAMauk17,Saur18,Damiano19:jupiter,Lysak21) generate field-aligned electron streams bouncing within the current sheet in the Jovian magnetodisk. These streams contribute to the field-aligned electron anisotropy, , and fire-hose parameter . In typical thick current sheets such anisotropy supports the cross-field electron currents (see Eq.(1)) and may create a thin, sub-ion scale current sheet embedded into a thick, ion scale current sheet [<]e.g.,¿Zelenyi04,Mingalev18,Kamaletdinov20:pop,Zelenyi22. Indeed, the magnetic field profiles in Fig. 5 exhibit stronger gradients around . If external drivers result in the current sheet thinning, the current sheet may reach sub-ion spatial scale where ions cannot redistribute their pressure and maintain the stress balance. In this case, the electron currents form peaks to balance the drop at the current sheet center, and self-consistently evolve from the cross-field currents to field-aligned currents (see Schematic in Fig. 1).
4 Discussion
In this study, we investigate force-free (and partially force-free) current sheets, where field-aligned electron streams support the pressure anisotropy and parallel currents, leading to the formation of the peak at the current sheet center, . Let us discuss the difference of the stress balance in such current sheets from that in more typical thick current sheets. In current sheets, the 2D stress balance in the equatorial plane (balance along the radial direction) is maintained by a combination of the centrifugal force, plasma pressure force, and magnetic field line tension force [Hill \BBA Carbary (\APACyear1978), Cheng (\APACyear1983), Zimbardo (\APACyear1989)]:
(3) |
where is the radial component of the plasma pressure tensor gradient. In the local coordinate system, and . Based on the vertical stress balance, , we may estimate the current density as:
(4) |
where and are the empirical relations [<]see, e.g.,¿Artemyev14:pss,Liu21:juno. The corresponding current sheet thickness should be larger than at , which is consistent with the thickness estimates for typical thick current sheets [<]e.g.,¿Khurana04,Liu21:juno. Such current sheets will be crossed during an interval of ; for much thinner current sheets as in our dataset, the traversal timescale will be less than min (see Fig. 3). The stress balance in such thin current sheets cannot be maintained by centrifugal force and radial gradient of the plasma pressure. Instead, the electron pressure anisotropy contributes to the stress balance [Rich \BOthers. (\APACyear1972)]:
(5) |
This equation shows that the thin current sheet configuration resembles a classical rotational discontinuity, with no variations of the Alfven speed because of the pressure anisotropy [Hudson (\APACyear1970)]:
(6) |
This condition allows for a balance of the 1D current sheet (with thickness much smaller than the spatial scale of the radial gradient of the plasma density) without fast plasma flows typical for rotational discontinuities in anisotropic plasma, where cross-sheet change of the plasma flow velocity equals to [<]see discussions of the anisotropy contribution to the force-free current sheet configurations in¿Vasko14:angeo_by,Artemyev20:apjl. Formation of such 1D current sheets around the fire-hose marginally stability threshold has been predicted theoretically [<]e.g.,¿FP76,Cowley78,Cowley&Pellat79, but never have been detected under quiet geomagnetic conditions in the Earth’s magnetotail [<]see discussion in¿Sitnov06,Artemyev&Zelenyi13. Observations of these current sheets in the Jovian magnetotail confirm the theoretical predictions, which can further lead to improved current sheet models [<]see discussion on development of the next generation of current sheet models in¿Sitnov&Merkin16,Yoon21:NatCom,Zelenyi22.
It is worth to note that these current sheets are electromagnetically disconnected from the Jovian ionosphere, because the local Alfven speed is zero, , and there are no field-aligned perturbations propagating from the current sheet to the ionosphere. Such local destruction of magnetosphere-ionosphere coupling is an interesting phenomenon that we do not observe in the Earth’s magnetotail, where is much more moderate [Artemyev, Angelopoulos, Vasko, Petrukovich\BCBL \BOthers. (\APACyear2020)].
Theoretical investigations of these force-free current sheets in the Jovian magnetodisk is a real challenge for plasma kinetics, because these current sheets share properties of 2D plasma equilibria (with the tension force ) and properties of rotational discontinuities (with and ). All existing 2D kinetic current sheet models operate with the plasma pressure gradients, , and do not include field-aligned currents [<]see, e.g.,¿[and references therein]YL05,Vasko13:pop,Zelenyi11PPR,Sitnov06,Sitnov&Merkin16. Existing models of force-free current sheets mostly assume 1D tangential discontinuities with [<]see, e.g.,¿[and references therein]Harrison09,Panov11:magnetopause,Neukirch20,Neukirch20:jpp. Construction of the kinetic model for 1D rotational discontinuities requires assumptions of an additional system symmetry [<]e.g.,¿Sonnerup&Su67,Artemyev11:pop,Mingalev12,Vasko14:angeo_by, whereas kinetic models of 2D rotational discontinuities have not yet been constructed. Although fluid models of 2D rotational discontinuities (with , , and ) can be constructed [<]e.g.,¿Cowley78,Hilmer87,Hau&Voigt92,Lukin18, their kinetic realization has not been demonstrated. Therefore, further theoretical investigations are needed to explain Juno observations in the Jovian magnetodisk.
5 Conclusions
We have investigated thin (thickness is smaller than or about the hot ion gyroradius) current sheets observed by Juno in the Jovian magnetodisk and characterized by strong field-aligned electron streams. We have demonstrated that electron streams support the strong field-aligned anisotropy, which may increase the fire-hose parameter up to the instability threshold, . In such thin, marginally stable current sheets, almost all current is field-aligned, and the current sheet configuration is force-free, with . This is a new type of strongly anisotropic, force-free current sheets, which has not been reported in the quiet-time Earth’s magnetopshere or solar wind. Further numerical investigations of such current sheet formation and dynamics will reveal their potential role in the particle acceleration (e.g., via magnetic reconnection).
Acknowledgements.
This work is supported by Grants 80NSSC19K1593 (A.V.A.) under Juno Participating Scientist program, and by subcontract 699046X to UCLA under prime contract ZZM06AA75C (X.J.Z. and Q.M.).Open Research
Data processing was done using SPEDAS V4.1, see \citeAAngelopoulos19. All the adopted data are available in the archive https://doi.org/10.5281/zenodo.7470240
References
- Allegrini \BOthers. (\APACyear2020) \APACinsertmetastarAllegrini20{APACrefauthors}Allegrini, F., Mauk, B., Clark, G., Gladstone, G\BPBIR., Hue, V., Kurth, W\BPBIS.\BDBLWilson, R\BPBIJ. \APACrefYearMonthDay2020\APACmonth04. \BBOQ\APACrefatitleEnergy Flux and Characteristic Energy of Electrons Over Jupiter’s Main Auroral Emission Energy Flux and Characteristic Energy of Electrons Over Jupiter’s Main Auroral Emission.\BBCQ \APACjournalVolNumPagesJournal of Geophysical Research (Space Physics)1254e27693. {APACrefDOI} 10.1029/2019JA027693 \PrintBackRefs\CurrentBib
- Allen \BOthers. (\APACyear2019) \APACinsertmetastarAllen19{APACrefauthors}Allen, R\BPBIC., Paranicas, C\BPBIP., Bagenal, F., Vines, S\BPBIK., Hamilton, D\BPBIC., Allegrini, F.\BDBLWilson, R\BPBIJ. \APACrefYearMonthDay2019Nov. \BBOQ\APACrefatitleEnergetic Oxygen and Sulfur Charge States in the Outer Jovian Magnetosphere: Insights From the Cassini Jupiter Flyby Energetic Oxygen and Sulfur Charge States in the Outer Jovian Magnetosphere: Insights From the Cassini Jupiter Flyby.\BBCQ \APACjournalVolNumPagesGeophys. Res. Lett.462111,709-11,717. {APACrefDOI} 10.1029/2019GL085185 \PrintBackRefs\CurrentBib
- Angelopoulos \BOthers. (\APACyear2019) \APACinsertmetastarAngelopoulos19{APACrefauthors}Angelopoulos, V., Cruce, P., Drozdov, A., Grimes, E\BPBIW., Hatzigeorgiu, N., King, D\BPBIA.\BDBLSchroeder, P. \APACrefYearMonthDay2019\APACmonth01. \BBOQ\APACrefatitleThe Space Physics Environment Data Analysis System (SPEDAS) The Space Physics Environment Data Analysis System (SPEDAS).\BBCQ \APACjournalVolNumPagesSpace Sci. Rev.2159. {APACrefDOI} 10.1007/s11214-018-0576-4 \PrintBackRefs\CurrentBib
- Artemyev (\APACyear2011) \APACinsertmetastarArtemyev11:pop{APACrefauthors}Artemyev, A\BPBIV. \APACrefYearMonthDay2011\APACmonth02. \BBOQ\APACrefatitleA model of one-dimensional current sheet with parallel currents and normal component of magnetic field A model of one-dimensional current sheet with parallel currents and normal component of magnetic field.\BBCQ \APACjournalVolNumPagesPhysics of Plasmas182022104. {APACrefDOI} 10.1063/1.3552141 \PrintBackRefs\CurrentBib
- Artemyev, Angelopoulos, Halekas\BCBL \BOthers. (\APACyear2017) \APACinsertmetastarArtemyev17:jgr:mars{APACrefauthors}Artemyev, A\BPBIV., Angelopoulos, V., Halekas, J\BPBIS., Runov, A., Zelenyi, L\BPBIM.\BCBL \BBA McFadden, J\BPBIP. \APACrefYearMonthDay2017\APACmonth05. \BBOQ\APACrefatitleMars’s magnetotail: Nature’s current sheet laboratory Mars’s magnetotail: Nature’s current sheet laboratory.\BBCQ \APACjournalVolNumPagesJ. Geophys. Res.1225404-5417. {APACrefDOI} 10.1002/2017JA024078 \PrintBackRefs\CurrentBib
- Artemyev, Angelopoulos, Liu\BCBL \BBA Runov (\APACyear2017) \APACinsertmetastarArtemyev17:grl:currents{APACrefauthors}Artemyev, A\BPBIV., Angelopoulos, V., Liu, J.\BCBL \BBA Runov, A. \APACrefYearMonthDay2017\APACmonth01. \BBOQ\APACrefatitleElectron currents supporting the near-Earth magnetotail during current sheet thinning Electron currents supporting the near-Earth magnetotail during current sheet thinning.\BBCQ \APACjournalVolNumPagesGeophys. Res. Lett.445-11. {APACrefDOI} 10.1002/2016GL072011 \PrintBackRefs\CurrentBib
- Artemyev, Angelopoulos, Vasko, Petrukovich\BCBL \BOthers. (\APACyear2020) \APACinsertmetastarArtemyev20:jgr:electrons{APACrefauthors}Artemyev, A\BPBIV., Angelopoulos, V., Vasko, I\BPBIY., Petrukovich, A\BPBIA., Runov, A., Saito, Y.\BDBLStrangeway, R\BPBIJ. \APACrefYearMonthDay2020\APACmonth01. \BBOQ\APACrefatitleContribution of Anisotropic Electron Current to the Magnetotail Current Sheet as a Function of Location and Plasma Conditions Contribution of Anisotropic Electron Current to the Magnetotail Current Sheet as a Function of Location and Plasma Conditions.\BBCQ \APACjournalVolNumPagesJournal of Geophysical Research (Space Physics)1251e27251. {APACrefDOI} 10.1029/2019JA027251 \PrintBackRefs\CurrentBib
- Artemyev, Angelopoulos, Vasko\BCBL \BBA Zelenyi (\APACyear2020) \APACinsertmetastarArtemyev20:apjl{APACrefauthors}Artemyev, A\BPBIV., Angelopoulos, V., Vasko, I\BPBIY.\BCBL \BBA Zelenyi, L\BPBIM. \APACrefYearMonthDay2020\APACmonth01. \BBOQ\APACrefatitleIon Nongyrotropy in Solar Wind Discontinuities Ion Nongyrotropy in Solar Wind Discontinuities.\BBCQ \APACjournalVolNumPagesApJ8891L23. {APACrefDOI} 10.3847/2041-8213/ab6b2e \PrintBackRefs\CurrentBib
- Artemyev \BOthers. (\APACyear2013) \APACinsertmetastarArtemyev13:jgr{APACrefauthors}Artemyev, A\BPBIV., Petrukovich, A\BPBIA., Frank, A\BPBIG., Nakamura, R.\BCBL \BBA Zelenyi, L\BPBIM. \APACrefYearMonthDay2013\APACmonth06. \BBOQ\APACrefatitleIntense current sheets in the magnetotail: Peculiarities of electron physics Intense current sheets in the magnetotail: Peculiarities of electron physics.\BBCQ \APACjournalVolNumPagesJ. Geophys. Res.1182789-2799. {APACrefDOI} 10.1002/jgra.50297 \PrintBackRefs\CurrentBib
- Artemyev \BOthers. (\APACyear2009) \APACinsertmetastarArtemyev09:angeo{APACrefauthors}Artemyev, A\BPBIV., Petrukovich, A\BPBIA., Zelenyi, L\BPBIM., Nakamura, R., Malova, H\BPBIV.\BCBL \BBA Popov, V\BPBIY. \APACrefYearMonthDay2009\APACmonth10. \BBOQ\APACrefatitleThin embedded current sheets: Cluster observations of ion kinetic structure and analytical models Thin embedded current sheets: Cluster observations of ion kinetic structure and analytical models.\BBCQ \APACjournalVolNumPagesAnnales Geophysicae274075-4087. \PrintBackRefs\CurrentBib
- Artemyev \BOthers. (\APACyear2016) \APACinsertmetastarArtemyev16:pop:cs{APACrefauthors}Artemyev, A\BPBIV., Vasko, I\BPBIY., Angelopoulos, V.\BCBL \BBA Runov, A. \APACrefYearMonthDay2016\APACmonth09. \BBOQ\APACrefatitleEffects of electron pressure anisotropy on current sheet configuration Effects of electron pressure anisotropy on current sheet configuration.\BBCQ \APACjournalVolNumPagesPhysics of Plasmas239092901. {APACrefDOI} 10.1063/1.4961926 \PrintBackRefs\CurrentBib
- Artemyev \BOthers. (\APACyear2014) \APACinsertmetastarArtemyev14:pss{APACrefauthors}Artemyev, A\BPBIV., Vasko, I\BPBIY.\BCBL \BBA Kasahara, S. \APACrefYearMonthDay2014. \BBOQ\APACrefatitleThin current sheets in the Jovian magnetotail. Thin current sheets in the Jovian magnetotail.\BBCQ \APACjournalVolNumPagesPlanet. Space Sci.96133-145. {APACrefDOI} 10.1016/j.pss.2014.03.012 \PrintBackRefs\CurrentBib
- Artemyev \BBA Zelenyi (\APACyear2013) \APACinsertmetastarArtemyev&Zelenyi13{APACrefauthors}Artemyev, A\BPBIV.\BCBT \BBA Zelenyi, L\BPBIM. \APACrefYearMonthDay2013. \BBOQ\APACrefatitleKinetic Structure of Current Sheets in the Earth Magnetotail Kinetic Structure of Current Sheets in the Earth Magnetotail.\BBCQ \APACjournalVolNumPagesSpace Sci. Rev.178419-440. {APACrefDOI} 10.1007/s11214-012-9954-5 \PrintBackRefs\CurrentBib
- Bagenal (\APACyear1992) \APACinsertmetastarBagenal92{APACrefauthors}Bagenal, F. \APACrefYearMonthDay1992. \BBOQ\APACrefatitleGiant planet magnetospheres Giant planet magnetospheres.\BBCQ \APACjournalVolNumPagesAnnual Review of Earth and Planetary Sciences20289-328. {APACrefDOI} 10.1146/annurev.ea.20.050192.001445 \PrintBackRefs\CurrentBib
- Bagenal \BOthers. (\APACyear2017) \APACinsertmetastarBagenal17{APACrefauthors}Bagenal, F., Adriani, A., Allegrini, F., Bolton, S\BPBIJ., Bonfond, B., Bunce, E\BPBIJ.\BDBLZarka, P. \APACrefYearMonthDay2017\APACmonth11. \BBOQ\APACrefatitleMagnetospheric Science Objectives of the Juno Mission Magnetospheric Science Objectives of the Juno Mission.\BBCQ \APACjournalVolNumPagesSpace Sci. Rev.213219-287. {APACrefDOI} 10.1007/s11214-014-0036-8 \PrintBackRefs\CurrentBib
- Bagenal \BBA Murdin (\APACyear2000) \APACinsertmetastarbookBagenal00{APACrefauthors}Bagenal, F.\BCBT \BBA Murdin, P. \APACrefYearMonthDay2000\APACmonth11. \BBOQ\APACrefatitlePlanetary Magnetospheres Planetary Magnetospheres.\BBCQ \BIn \APACrefbtitleEncyclopedia of Astronomy and Astrophysics. Encyclopedia of astronomy and astrophysics. {APACrefDOI} 10.1888/0333750888/2322 \PrintBackRefs\CurrentBib
- Birn \BOthers. (\APACyear2012) \APACinsertmetastarBirn12:SSR{APACrefauthors}Birn, J., Artemyev, A\BPBIV., Baker, D\BPBIN., Echim, M., Hoshino, M.\BCBL \BBA Zelenyi, L\BPBIM. \APACrefYearMonthDay2012. \BBOQ\APACrefatitleParticle acceleration in the magnetotail and aurora Particle acceleration in the magnetotail and aurora.\BBCQ \APACjournalVolNumPagesSpace Sci. Rev.17349-102. {APACrefDOI} 10.1007/s11214-012-9874-4 \PrintBackRefs\CurrentBib
- Birn, Dorelli\BCBL \BOthers. (\APACyear2004) \APACinsertmetastarBirn04MHD{APACrefauthors}Birn, J., Dorelli, J\BPBIC., Hesse, M.\BCBL \BBA Schindler, K. \APACrefYearMonthDay2004\APACmonth02. \BBOQ\APACrefatitleThin current sheets and loss of equilibrium: Three-dimensional theory and simulations Thin current sheets and loss of equilibrium: Three-dimensional theory and simulations.\BBCQ \APACjournalVolNumPagesJ. Geophys. Res.1092215. {APACrefDOI} 10.1029/2003JA010275 \PrintBackRefs\CurrentBib
- Birn, Schindler\BCBL \BBA Hesse (\APACyear2004) \APACinsertmetastarBirn04{APACrefauthors}Birn, J., Schindler, K.\BCBL \BBA Hesse, M. \APACrefYearMonthDay2004\APACmonth02. \BBOQ\APACrefatitleThin electron current sheets and their relation to auroral potentials Thin electron current sheets and their relation to auroral potentials.\BBCQ \APACjournalVolNumPagesJ. Geophys. Res.1092217. {APACrefDOI} 10.1029/2003JA010303 \PrintBackRefs\CurrentBib
- Chaston \BOthers. (\APACyear2007) \APACinsertmetastarChaston07{APACrefauthors}Chaston, C\BPBIC., Carlson, C\BPBIW., McFadden, J\BPBIP., Ergun, R\BPBIE.\BCBL \BBA Strangeway, R\BPBIJ. \APACrefYearMonthDay2007\APACmonth04. \BBOQ\APACrefatitleHow important are dispersive Alfvén waves for auroral particle acceleration? How important are dispersive Alfvén waves for auroral particle acceleration?\BBCQ \APACjournalVolNumPagesGeophys. Res. Lett.347L07101. {APACrefDOI} 10.1029/2006GL029144 \PrintBackRefs\CurrentBib
- Cheng (\APACyear1983) \APACinsertmetastarCheng83{APACrefauthors}Cheng, A\BPBIF. \APACrefYearMonthDay1983\APACmonth01. \BBOQ\APACrefatitleThin, rotating plasma disks Thin, rotating plasma disks.\BBCQ \APACjournalVolNumPagesJ. Geophys. Res.8813-18. {APACrefDOI} 10.1029/JA088iA01p00013 \PrintBackRefs\CurrentBib
- Clark \BOthers. (\APACyear2016) \APACinsertmetastarClark16{APACrefauthors}Clark, G., Mauk, B\BPBIH., Paranicas, C., Kollmann, P.\BCBL \BBA Smith, H\BPBIT. \APACrefYearMonthDay2016Mar. \BBOQ\APACrefatitleCharge states of energetic oxygen and sulfur ions in Jupiter’s magnetosphere Charge states of energetic oxygen and sulfur ions in Jupiter’s magnetosphere.\BBCQ \APACjournalVolNumPagesJournal of Geophysical Research (Space Physics)12132264-2273. {APACrefDOI} 10.1002/2015JA022257 \PrintBackRefs\CurrentBib
- Connerney \BOthers. (\APACyear1998) \APACinsertmetastarConnerney98{APACrefauthors}Connerney, J\BPBIE\BPBIP., Acuña, M\BPBIH., Ness, N\BPBIF.\BCBL \BBA Satoh, T. \APACrefYearMonthDay1998Jun. \BBOQ\APACrefatitleNew models of Jupiter’s magnetic field constrained by the Io flux tube footprint New models of Jupiter’s magnetic field constrained by the Io flux tube footprint.\BBCQ \APACjournalVolNumPagesJ. Geophys. Res.103A611929-11940. {APACrefDOI} 10.1029/97JA03726 \PrintBackRefs\CurrentBib
- Connerney, Adriani\BCBL \BOthers. (\APACyear2017) \APACinsertmetastarConnerney17:science{APACrefauthors}Connerney, J\BPBIE\BPBIP., Adriani, A., Allegrini, F., Bagenal, F., Bolton, S\BPBIJ., Bonfond, B.\BDBLWaite, J. \APACrefYearMonthDay2017\APACmonth05. \BBOQ\APACrefatitleJupiter’s magnetosphere and aurorae observed by the Juno spacecraft during its first polar orbits Jupiter’s magnetosphere and aurorae observed by the Juno spacecraft during its first polar orbits.\BBCQ \APACjournalVolNumPagesScience356826-832. {APACrefDOI} 10.1126/science.aam5928 \PrintBackRefs\CurrentBib
- Connerney, Benn\BCBL \BOthers. (\APACyear2017) \APACinsertmetastarConnerney17:ssr{APACrefauthors}Connerney, J\BPBIE\BPBIP., Benn, M., Bjarno, J\BPBIB., Denver, T., Espley, J., Jorgensen, J\BPBIL.\BDBLSmith, E\BPBIJ. \APACrefYearMonthDay2017\APACmonth11. \BBOQ\APACrefatitleThe Juno Magnetic Field Investigation The Juno Magnetic Field Investigation.\BBCQ \APACjournalVolNumPagesSpace Sci. Rev.21339-138. {APACrefDOI} 10.1007/s11214-017-0334-z \PrintBackRefs\CurrentBib
- Connerney \BOthers. (\APACyear2020) \APACinsertmetastarConnerney20:Magnetodisc_Model{APACrefauthors}Connerney, J\BPBIE\BPBIP., Timmins, S., Herceg, M.\BCBL \BBA Joergensen, J\BPBIL. \APACrefYearMonthDay2020\APACmonth10. \BBOQ\APACrefatitleA Jovian Magnetodisc Model for the Juno Era A Jovian Magnetodisc Model for the Juno Era.\BBCQ \APACjournalVolNumPagesJournal of Geophysical Research (Space Physics)12510e28138. {APACrefDOI} 10.1029/2020JA028138 \PrintBackRefs\CurrentBib
- Cowley (\APACyear1978) \APACinsertmetastarCowley78{APACrefauthors}Cowley, S\BPBIW\BPBIH. \APACrefYearMonthDay1978\APACmonth11. \BBOQ\APACrefatitleThe effect of pressure anisotropy on the equilibrium structure of magnetic current sheets The effect of pressure anisotropy on the equilibrium structure of magnetic current sheets.\BBCQ \APACjournalVolNumPagesPlanetary and Space Science261037-1061. {APACrefDOI} 10.1016/0032-0633(78)90028-4 \PrintBackRefs\CurrentBib
- Cowley \BBA Pellat (\APACyear1979) \APACinsertmetastarCowley&Pellat79{APACrefauthors}Cowley, S\BPBIW\BPBIH.\BCBT \BBA Pellat, R. \APACrefYearMonthDay1979\APACmonth03. \BBOQ\APACrefatitleA note on adiabatic solutions of the one-dimensional current sheet problem A note on adiabatic solutions of the one-dimensional current sheet problem.\BBCQ \APACjournalVolNumPagesPlanet. Space Sci.27265-271. {APACrefDOI} 10.1016/0032-0633(79)90069-2 \PrintBackRefs\CurrentBib
- Damiano \BOthers. (\APACyear2019) \APACinsertmetastarDamiano19:jupiter{APACrefauthors}Damiano, P\BPBIA., Delamere, P\BPBIA., Stauffer, B., Ng, C\BPBIS.\BCBL \BBA Johnson, J\BPBIR. \APACrefYearMonthDay2019\APACmonth03. \BBOQ\APACrefatitleKinetic Simulations of Electron Acceleration by Dispersive Scale Alfvén Waves in Jupiter’s Magnetosphere Kinetic Simulations of Electron Acceleration by Dispersive Scale Alfvén Waves in Jupiter’s Magnetosphere.\BBCQ \APACjournalVolNumPagesGeophys. Res. Lett.4663043-3051. {APACrefDOI} 10.1029/2018GL081219 \PrintBackRefs\CurrentBib
- DiBraccio \BOthers. (\APACyear2015) \APACinsertmetastarDiBraccio15{APACrefauthors}DiBraccio, G\BPBIA., Espley, J\BPBIR., Gruesbeck, J\BPBIR., Connerney, J\BPBIE\BPBIP., Brain, D\BPBIA., Halekas, J\BPBIS.\BDBLJakosky, B\BPBIM. \APACrefYearMonthDay2015\APACmonth11. \BBOQ\APACrefatitleMagnetotail dynamics at Mars: Initial MAVEN observations Magnetotail dynamics at Mars: Initial MAVEN observations.\BBCQ \APACjournalVolNumPagesGeophys. Res. Lett.428828-8837. {APACrefDOI} 10.1002/2015GL065248 \PrintBackRefs\CurrentBib
- Drake \BBA Lee (\APACyear1977) \APACinsertmetastarDrake&Lee77{APACrefauthors}Drake, J\BPBIF.\BCBT \BBA Lee, Y\BPBIC. \APACrefYearMonthDay1977\APACmonth08. \BBOQ\APACrefatitleKinetic theory of tearing instabilities Kinetic theory of tearing instabilities.\BBCQ \APACjournalVolNumPagesPhysics of Fluids201341-1353. {APACrefDOI} 10.1063/1.862017 \PrintBackRefs\CurrentBib
- Elliott \BOthers. (\APACyear2020) \APACinsertmetastarElliott20{APACrefauthors}Elliott, S\BPBIS., Gurnett, D\BPBIA., Yoon, P\BPBIH., Kurth, W\BPBIS., Mauk, B\BPBIH., Ebert, R\BPBIW.\BDBLSulaiman, A\BPBIH. \APACrefYearMonthDay2020\APACmonth06. \BBOQ\APACrefatitleThe Generation of Upward-Propagating Whistler Mode Waves by Electron Beams in the Jovian Polar Regions The Generation of Upward-Propagating Whistler Mode Waves by Electron Beams in the Jovian Polar Regions.\BBCQ \APACjournalVolNumPagesJournal of Geophysical Research (Space Physics)1256e27868. {APACrefDOI} 10.1029/2020JA027868 \PrintBackRefs\CurrentBib
- Ergun \BOthers. (\APACyear2004) \APACinsertmetastarErgun04{APACrefauthors}Ergun, R\BPBIE., Andersson, L., Main, D., Su, Y\BPBIJ., Newman, D\BPBIL., Goldman, M\BPBIV.\BDBLMozer, F\BPBIS. \APACrefYearMonthDay2004\APACmonth12. \BBOQ\APACrefatitleAuroral particle acceleration by strong double layers: The upward current region Auroral particle acceleration by strong double layers: The upward current region.\BBCQ \APACjournalVolNumPagesJournal of Geophysical Research (Space Physics)109A12A12220. {APACrefDOI} 10.1029/2004JA010545 \PrintBackRefs\CurrentBib
- Francfort \BBA Pellat (\APACyear1976) \APACinsertmetastarFP76{APACrefauthors}Francfort, P.\BCBT \BBA Pellat, R. \APACrefYearMonthDay1976\APACmonth08. \BBOQ\APACrefatitleMagnetic merging in collisionless plasmas Magnetic merging in collisionless plasmas.\BBCQ \APACjournalVolNumPagesGeophys. Res. Lett.3433-436. {APACrefDOI} 10.1029/GL003i008p00433 \PrintBackRefs\CurrentBib
- Frank \BOthers. (\APACyear2002) \APACinsertmetastarFrank02Jupiter{APACrefauthors}Frank, L\BPBIA., Paterson, W\BPBIR.\BCBL \BBA Khurana, K\BPBIK. \APACrefYearMonthDay2002\APACmonth01. \BBOQ\APACrefatitleObservations of thermal plasmas in Jupiter’s magnetotail Observations of thermal plasmas in Jupiter’s magnetotail.\BBCQ \APACjournalVolNumPagesJ. Geophys. Res.1071003. {APACrefDOI} 10.1029/2001JA000077 \PrintBackRefs\CurrentBib
- Gonzalez \BBA Parker (\APACyear2016) \APACinsertmetastarbook:Gonzalez&Parker{APACrefauthors}Gonzalez, W.\BCBT \BBA Parker, E. \APACrefYear2016. \APACrefbtitleMagnetic Reconnection Magnetic Reconnection (\BVOL 427). {APACrefDOI} 10.1007/978-3-319-26432-5 \PrintBackRefs\CurrentBib
- Hada \BOthers. (\APACyear1981) \APACinsertmetastarHada81{APACrefauthors}Hada, T., Nishida, A., Terasawa, T.\BCBL \BBA Hones, E\BPBIW., Jr. \APACrefYearMonthDay1981\APACmonth12. \BBOQ\APACrefatitleBi-directional electron pitch angle anisotropy in the plasma sheet Bi-directional electron pitch angle anisotropy in the plasma sheet.\BBCQ \APACjournalVolNumPagesJ. Geophys. Res.8611211-11224. {APACrefDOI} 10.1029/JA086iA13p11211 \PrintBackRefs\CurrentBib
- Harrison \BBA Neukirch (\APACyear2009) \APACinsertmetastarHarrison09{APACrefauthors}Harrison, M\BPBIG.\BCBT \BBA Neukirch, T. \APACrefYearMonthDay2009\APACmonth02. \BBOQ\APACrefatitleSome remarks on one-dimensional force-free Vlasov-Maxwell equilibria Some remarks on one-dimensional force-free Vlasov-Maxwell equilibria.\BBCQ \APACjournalVolNumPagesPhysics of Plasmas162022106. {APACrefDOI} 10.1063/1.3077307 \PrintBackRefs\CurrentBib
- Hau \BBA Voigt (\APACyear1992) \APACinsertmetastarHau&Voigt92{APACrefauthors}Hau, L\BPBIN.\BCBT \BBA Voigt, G\BPBIH. \APACrefYearMonthDay1992\APACmonth06. \BBOQ\APACrefatitleLoss of MHD equilibrium caused by the enhancment of the magnetic By component in Earth’s magnetotail Loss of MHD equilibrium caused by the enhancment of the magnetic By component in Earth’s magnetotail.\BBCQ \APACjournalVolNumPagesJ. Geophys. Res.97A68707-8711. {APACrefDOI} 10.1029/92JA00445 \PrintBackRefs\CurrentBib
- Hesse \BOthers. (\APACyear1998) \APACinsertmetastarHesse98{APACrefauthors}Hesse, M., Winske, D.\BCBL \BBA Birn, J. \APACrefYearMonthDay1998\APACmonth01. \BBOQ\APACrefatitleOn the ion-scale structure of thin current sheets in the magnetotail On the ion-scale structure of thin current sheets in the magnetotail.\BBCQ \APACjournalVolNumPagesPhysica Scripta Volume T7463-66. {APACrefDOI} 10.1088/0031-8949/1998/T74/012 \PrintBackRefs\CurrentBib
- Hill (\APACyear1979) \APACinsertmetastarHill79{APACrefauthors}Hill, T\BPBIW. \APACrefYearMonthDay1979\APACmonth11. \BBOQ\APACrefatitleInertial limit on corotation Inertial limit on corotation.\BBCQ \APACjournalVolNumPagesJ. Geophys. Res.846554-6558. {APACrefDOI} 10.1029/JA084iA11p06554 \PrintBackRefs\CurrentBib
- Hill \BBA Carbary (\APACyear1978) \APACinsertmetastarHill&Carbary78{APACrefauthors}Hill, T\BPBIW.\BCBT \BBA Carbary, J\BPBIF. \APACrefYearMonthDay1978Dec. \BBOQ\APACrefatitleCentrifugal distortion of the Jovian magnetosphere by an equatorially confined current sheet Centrifugal distortion of the Jovian magnetosphere by an equatorially confined current sheet.\BBCQ \APACjournalVolNumPagesJ. Geophys. Res.83A125745-5749. {APACrefDOI} 10.1029/JA083iA12p05745 \PrintBackRefs\CurrentBib
- Hilmer \BBA Voigt (\APACyear1987) \APACinsertmetastarHilmer87{APACrefauthors}Hilmer, R\BPBIV.\BCBT \BBA Voigt, G. \APACrefYearMonthDay1987\APACmonth08. \BBOQ\APACrefatitleThe effects of magnetic B(y) component on geomagnetic tail equilibria The effects of magnetic B(y) component on geomagnetic tail equilibria.\BBCQ \APACjournalVolNumPagesJ. Geophys. Res.928660-8672. {APACrefDOI} 10.1029/JA092iA08p08660 \PrintBackRefs\CurrentBib
- Hsieh \BBA Otto (\APACyear2015) \APACinsertmetastarHsieh&Otto15{APACrefauthors}Hsieh, M\BHBIS.\BCBT \BBA Otto, A. \APACrefYearMonthDay2015\APACmonth06. \BBOQ\APACrefatitleThin current sheet formation in response to the loading and the depletion of magnetic flux during the substorm growth phase Thin current sheet formation in response to the loading and the depletion of magnetic flux during the substorm growth phase.\BBCQ \APACjournalVolNumPagesJ. Geophys. Res.1204264-4278. {APACrefDOI} 10.1002/2014JA020925 \PrintBackRefs\CurrentBib
- Hudson (\APACyear1970) \APACinsertmetastarHudson70{APACrefauthors}Hudson, P\BPBID. \APACrefYearMonthDay1970\APACmonth11. \BBOQ\APACrefatitleDiscontinuities in an anisotropic plasma and their identification in the solar wind Discontinuities in an anisotropic plasma and their identification in the solar wind.\BBCQ \APACjournalVolNumPagesPlanet. Space Sci.181611-1622. {APACrefDOI} 10.1016/0032-0633(70)90036-X \PrintBackRefs\CurrentBib
- Huscher \BOthers. (\APACyear2021) \APACinsertmetastarBagenal21:Huscher_plasmasheet{APACrefauthors}Huscher, E., Bagenal, F., Wilson, R\BPBIJ., Allegrini, F., Ebert, R\BPBIW., Valek, P\BPBIW.\BDBLLevin, S\BPBIM. \APACrefYearMonthDay2021\APACmonth08. \BBOQ\APACrefatitleSurvey of Juno Observations in Jupiter’s Plasma Disk: Density Survey of Juno Observations in Jupiter’s Plasma Disk: Density.\BBCQ \APACjournalVolNumPagesJournal of Geophysical Research (Space Physics)1268e29446. {APACrefDOI} 10.1029/2021JA029446 \PrintBackRefs\CurrentBib
- Jackman \BOthers. (\APACyear2014) \APACinsertmetastarJackman14{APACrefauthors}Jackman, C\BPBIM., Arridge, C\BPBIS., André, N., Bagenal, F., Birn, J., Freeman, M\BPBIP.\BDBLWalsh, A\BPBIP. \APACrefYearMonthDay2014\APACmonth08. \BBOQ\APACrefatitleLarge-Scale Structure and Dynamics of the Magnetotails of Mercury, Earth, Jupiter and Saturn Large-Scale Structure and Dynamics of the Magnetotails of Mercury, Earth, Jupiter and Saturn.\BBCQ \APACjournalVolNumPagesSpace Sci. Rev.18285-154. {APACrefDOI} 10.1007/s11214-014-0060-8 \PrintBackRefs\CurrentBib
- Kamaletdinov \BOthers. (\APACyear2020) \APACinsertmetastarKamaletdinov20:pop{APACrefauthors}Kamaletdinov, S\BPBIR., Yushkov, E\BPBIV., Artemyev, A\BPBIV., Lukin, A\BPBIS.\BCBL \BBA Vasko, I\BPBIY. \APACrefYearMonthDay2020\APACmonth08. \BBOQ\APACrefatitleSuperthin current sheets supported by anisotropic electrons Superthin current sheets supported by anisotropic electrons.\BBCQ \APACjournalVolNumPagesPhysics of Plasmas278082904. {APACrefDOI} 10.1063/5.0018063 \PrintBackRefs\CurrentBib
- Kane \BOthers. (\APACyear1999) \APACinsertmetastarKane99{APACrefauthors}Kane, M., Williams, D\BPBIJ., Mauk, B\BPBIH., McEntire, R\BPBIW.\BCBL \BBA Roelof, E\BPBIC. \APACrefYearMonthDay1999\APACmonth01. \BBOQ\APACrefatitleGalileo Energetic Particles Detector measurements of hot ions in the neutral sheet region of Jupiter’s magnetodisk Galileo Energetic Particles Detector measurements of hot ions in the neutral sheet region of Jupiter’s magnetodisk.\BBCQ \APACjournalVolNumPagesGeophys. Res. Lett.265-8. {APACrefDOI} 10.1029/1998GL900267 \PrintBackRefs\CurrentBib
- Khurana \BOthers. (\APACyear2004) \APACinsertmetastarKhurana04{APACrefauthors}Khurana, K\BPBIK., Kivelson, M\BPBIG., Vasyliunas, V\BPBIM., Krupp, N., Woch, J., Lagg, A.\BDBLKurth, W\BPBIS. \APACrefYearMonthDay2004. \BBOQ\APACrefatitleThe configuration of Jupiter’s magnetosphere The configuration of Jupiter’s magnetosphere.\BBCQ \BIn F. Bagenal, T\BPBIE. Dowling\BCBL \BBA W\BPBIB. McKinnon (\BEDS), \APACrefbtitleJupiter. The Planet, Satellites and Magnetosphere Jupiter. the planet, satellites and magnetosphere (\BPG 593-616). \PrintBackRefs\CurrentBib
- Khurana \BOthers. (\APACyear2022) \APACinsertmetastarKhurana22{APACrefauthors}Khurana, K\BPBIK., Leinweber, H\BPBIK., Hospodarsky, G\BPBIB.\BCBL \BBA Paranicas, C\BPBIP. \APACrefYearMonthDay2022. \BBOQ\APACrefatitleRadial and Local Time Variations in the Thickness of Jupiter’s Magnetospheric Current Sheet Radial and local time variations in the thickness of jupiter’s magnetospheric current sheet.\BBCQ \APACjournalVolNumPagesJournal of Geophysical Research: Space Physics12710e2022JA030664. {APACrefDOI} doi:10.1029/2022JA030664 \PrintBackRefs\CurrentBib
- Khurana \BBA Liu (\APACyear2018) \APACinsertmetastarKhurana&Liu18{APACrefauthors}Khurana, K\BPBIK.\BCBT \BBA Liu, J. \APACrefYearMonthDay2018\APACmonth03. \BBOQ\APACrefatitleCurrent Systems in Planetary Magnetospheres: A Comparative Overview Current Systems in Planetary Magnetospheres: A Comparative Overview.\BBCQ \BIn A. Keiling, O. Marghitu\BCBL \BBA M. Wheatland (\BEDS), \APACrefbtitleElectric Currents in Geospace and Beyond Electric currents in geospace and beyond (\BVOL 235, \BPG 17-41). {APACrefDOI} 10.1002/9781119324522.ch2 \PrintBackRefs\CurrentBib
- Khurana \BBA Schwarzl (\APACyear2005) \APACinsertmetastarKhurana05{APACrefauthors}Khurana, K\BPBIK.\BCBT \BBA Schwarzl, H\BPBIK. \APACrefYearMonthDay2005\APACmonth07. \BBOQ\APACrefatitleGlobal structure of Jupiter’s magnetospheric current sheet Global structure of Jupiter’s magnetospheric current sheet.\BBCQ \APACjournalVolNumPagesJ. Geophys. Res.1107227. {APACrefDOI} 10.1029/2004JA010757 \PrintBackRefs\CurrentBib
- Kim \BOthers. (\APACyear2020\APACexlab\BCnt1) \APACinsertmetastarKim20:juno{APACrefauthors}Kim, T\BPBIK., Ebert, R\BPBIW., Valek, P\BPBIW., Allegrini, F., McComas, D\BPBIJ., Bagenal, F.\BDBLNicolaou, G. \APACrefYearMonthDay2020\BCnt1\APACmonth02. \BBOQ\APACrefatitleMethod to Derive Ion Properties From Juno JADE Including Abundance Estimates for O+ and S2+ Method to Derive Ion Properties From Juno JADE Including Abundance Estimates for O+ and S2+.\BBCQ \APACjournalVolNumPagesJournal of Geophysical Research (Space Physics)1252e26169. {APACrefDOI} 10.1029/2018JA026169 \PrintBackRefs\CurrentBib
- Kim \BOthers. (\APACyear2020\APACexlab\BCnt2) \APACinsertmetastarKim20:juno:PS{APACrefauthors}Kim, T\BPBIK., Ebert, R\BPBIW., Valek, P\BPBIW., Allegrini, F., McComas, D\BPBIJ., Bagenal, F.\BDBLBolton, S\BPBIJ. \APACrefYearMonthDay2020\BCnt2\APACmonth04. \BBOQ\APACrefatitleSurvey of Ion Properties in Jupiter’s Plasma Sheet: Juno JADE-I Observations Survey of Ion Properties in Jupiter’s Plasma Sheet: Juno JADE-I Observations.\BBCQ \APACjournalVolNumPagesJournal of Geophysical Research (Space Physics)1254e27696. {APACrefDOI} 10.1029/2019JA027696 \PrintBackRefs\CurrentBib
- Kollmann \BOthers. (\APACyear2018) \APACinsertmetastarKollmann18{APACrefauthors}Kollmann, P., Roussos, E., Paranicas, C., Woodfield, E\BPBIE., Mauk, B\BPBIH., Clark, G.\BDBLVandegriff, J. \APACrefYearMonthDay2018\APACmonth11. \BBOQ\APACrefatitleElectron Acceleration to MeV Energies at Jupiter and Saturn Electron Acceleration to MeV Energies at Jupiter and Saturn.\BBCQ \APACjournalVolNumPagesJournal of Geophysical Research (Space Physics)123119110-9129. {APACrefDOI} 10.1029/2018JA025665 \PrintBackRefs\CurrentBib
- Kronberg \BOthers. (\APACyear2012) \APACinsertmetastarKronberg12:icarus{APACrefauthors}Kronberg, E\BPBIA., Kasahara, S., Krupp, N.\BCBL \BBA Woch, J. \APACrefYearMonthDay2012\APACmonth01. \BBOQ\APACrefatitleField-aligned beams and reconnection in the jovian magnetotail Field-aligned beams and reconnection in the jovian magnetotail.\BBCQ \APACjournalVolNumPagesIcarus21755-65. {APACrefDOI} 10.1016/j.icarus.2011.10.011 \PrintBackRefs\CurrentBib
- Krupp \BOthers. (\APACyear2004) \APACinsertmetastarKrupp04{APACrefauthors}Krupp, N., Woch, J., Lagg, A., Livi, S., Mitchell, D\BPBIG., Krimigis, S\BPBIM.\BDBLEspinosa, S\BPBIA. \APACrefYearMonthDay2004Sep. \BBOQ\APACrefatitleEnergetic particle observations in the vicinity of Jupiter: Cassini MIMI/LEMMS results Energetic particle observations in the vicinity of Jupiter: Cassini MIMI/LEMMS results.\BBCQ \APACjournalVolNumPagesJournal of Geophysical Research (Space Physics)109A9A09S10. {APACrefDOI} 10.1029/2003JA010111 \PrintBackRefs\CurrentBib
- Liu \BOthers. (\APACyear2021) \APACinsertmetastarLiu21:juno{APACrefauthors}Liu, Z\BPBIY., Zong, Q\BPBIG., Blanc, M., Sun, Y\BPBIX., Zhao, J\BPBIT., Hao, Y\BPBIX.\BCBL \BBA Mauk, B\BPBIH. \APACrefYearMonthDay2021\APACmonth11. \BBOQ\APACrefatitleStatistics on Jupiter’s Current Sheet With Juno Data: Geometry, Magnetic Fields and Energetic Particles Statistics on Jupiter’s Current Sheet With Juno Data: Geometry, Magnetic Fields and Energetic Particles.\BBCQ \APACjournalVolNumPagesJournal of Geophysical Research (Space Physics)12611e29710. {APACrefDOI} 10.1029/2021JA029710 \PrintBackRefs\CurrentBib
- Lu \BOthers. (\APACyear2019) \APACinsertmetastarLu19:jgr:cs{APACrefauthors}Lu, S., Artemyev, A\BPBIV., Angelopoulos, V., Lin, Y., Zhang, X\BPBIJ., Liu, J.\BDBLStrangeway, R\BPBIJ. \APACrefYearMonthDay2019Feb. \BBOQ\APACrefatitleThe Hall Electric Field in Earth’s Magnetotail Thin Current Sheet The Hall Electric Field in Earth’s Magnetotail Thin Current Sheet.\BBCQ \APACjournalVolNumPagesJournal of Geophysical Research (Space Physics)12421052-1062. {APACrefDOI} 10.1029/2018JA026202 \PrintBackRefs\CurrentBib
- Lu \BOthers. (\APACyear2016) \APACinsertmetastarLu16:CS{APACrefauthors}Lu, S., Lin, Y., Angelopoulos, V., Artemyev, A\BPBIV., Pritchett, P\BPBIL., Lu, Q.\BCBL \BBA Wang, X\BPBIY. \APACrefYearMonthDay2016\APACmonth12. \BBOQ\APACrefatitleHall effect control of magnetotail dawn-dusk asymmetry: A three-dimensional global hybrid simulation Hall effect control of magnetotail dawn-dusk asymmetry: A three-dimensional global hybrid simulation.\BBCQ \APACjournalVolNumPagesJ. Geophys. Res.12111. {APACrefDOI} 10.1002/2016JA023325 \PrintBackRefs\CurrentBib
- Lukin \BOthers. (\APACyear2018) \APACinsertmetastarLukin18{APACrefauthors}Lukin, A\BPBIS., Vasko, I., Artemyev, A.\BCBL \BBA Yushkov, E. \APACrefYearMonthDay2018\APACmonth01. \BBOQ\APACrefatitleTwo-dimensional self-similar plasma equilibria Two-dimensional self-similar plasma equilibria.\BBCQ \APACjournalVolNumPagesPhysics of Plasmas251012906. {APACrefDOI} 10.1063/1.5016178 \PrintBackRefs\CurrentBib
- Lysak \BOthers. (\APACyear2021) \APACinsertmetastarLysak21{APACrefauthors}Lysak, R\BPBIL., Song, Y., Elliott, S., Kurth, W., Sulaiman, A\BPBIH.\BCBL \BBA Gershman, D. \APACrefYearMonthDay2021\APACmonth12. \BBOQ\APACrefatitleThe Jovian Ionospheric Alfvén Resonator and Auroral Particle Acceleration The Jovian Ionospheric Alfvén Resonator and Auroral Particle Acceleration.\BBCQ \APACjournalVolNumPagesJournal of Geophysical Research (Space Physics)12612e29886. {APACrefDOI} 10.1029/2021JA029886 \PrintBackRefs\CurrentBib
- Mauk \BOthers. (\APACyear2020) \APACinsertmetastarMauk20{APACrefauthors}Mauk, B\BPBIH., Clark, G., Gladstone, G\BPBIR., Kotsiaros, S., Adriani, A., Allegrini, F.\BDBLRymer, A\BPBIM. \APACrefYearMonthDay2020\APACmonth03. \BBOQ\APACrefatitleEnergetic Particles and Acceleration Regions Over Jupiter’s Polar Cap and Main Aurora: A Broad Overview Energetic Particles and Acceleration Regions Over Jupiter’s Polar Cap and Main Aurora: A Broad Overview.\BBCQ \APACjournalVolNumPagesJournal of Geophysical Research (Space Physics)1253e27699. {APACrefDOI} 10.1029/2019JA027699 \PrintBackRefs\CurrentBib
- Mauk \BOthers. (\APACyear2017\APACexlab\BCnt1) \APACinsertmetastarMauk17:nature{APACrefauthors}Mauk, B\BPBIH., Haggerty, D\BPBIK., Paranicas, C., Clark, G., Kollmann, P., Rymer, A\BPBIM.\BDBLValek, P. \APACrefYearMonthDay2017\BCnt1\APACmonth09. \BBOQ\APACrefatitleDiscrete and broadband electron acceleration in Jupiter’s powerful aurora Discrete and broadband electron acceleration in Jupiter’s powerful aurora.\BBCQ \APACjournalVolNumPagesNature549767066-69. {APACrefDOI} 10.1038/nature23648 \PrintBackRefs\CurrentBib
- Mauk \BOthers. (\APACyear2017\APACexlab\BCnt2) \APACinsertmetastarMauk17{APACrefauthors}Mauk, B\BPBIH., Haggerty, D\BPBIK., Paranicas, C., Clark, G., Kollmann, P., Rymer, A\BPBIM.\BDBLValek, P. \APACrefYearMonthDay2017\BCnt2\APACmonth05. \BBOQ\APACrefatitleJuno observations of energetic charged particles over Jupiter’s polar regions: Analysis of monodirectional and bidirectional electron beams Juno observations of energetic charged particles over Jupiter’s polar regions: Analysis of monodirectional and bidirectional electron beams.\BBCQ \APACjournalVolNumPagesGeophys. Res. Lett.444410-4418. {APACrefDOI} 10.1002/2016GL072286 \PrintBackRefs\CurrentBib
- Mauk \BOthers. (\APACyear2004) \APACinsertmetastarMauk04{APACrefauthors}Mauk, B\BPBIH., Mitchell, D\BPBIG., McEntire, R\BPBIW., Paranicas, C\BPBIP., Roelof, E\BPBIC., Williams, D\BPBIJ.\BDBLLagg, A. \APACrefYearMonthDay2004\APACmonth07. \BBOQ\APACrefatitleEnergetic ion characteristics and neutral gas interactions in Jupiter’s magnetosphere Energetic ion characteristics and neutral gas interactions in Jupiter’s magnetosphere.\BBCQ \APACjournalVolNumPagesJ. Geophys. Res.1099. {APACrefDOI} 10.1029/2003JA010270 \PrintBackRefs\CurrentBib
- McComas, Alexander\BCBL \BOthers. (\APACyear2017) \APACinsertmetastarMcComas17:ssr{APACrefauthors}McComas, D\BPBIJ., Alexander, N., Allegrini, F., Bagenal, F., Beebe, C., Clark, G.\BDBLWhite, D. \APACrefYearMonthDay2017\APACmonth11. \BBOQ\APACrefatitleThe Jovian Auroral Distributions Experiment (JADE) on the Juno Mission to Jupiter The Jovian Auroral Distributions Experiment (JADE) on the Juno Mission to Jupiter.\BBCQ \APACjournalVolNumPagesSpace Sci. Rev.213547-643. {APACrefDOI} 10.1007/s11214-013-9990-9 \PrintBackRefs\CurrentBib
- McComas, Szalay\BCBL \BOthers. (\APACyear2017) \APACinsertmetastarMcComas17{APACrefauthors}McComas, D\BPBIJ., Szalay, J\BPBIR., Allegrini, F., Bagenal, F., Connerney, J., Ebert, R\BPBIW.\BDBLBolton, S. \APACrefYearMonthDay2017\APACmonth05. \BBOQ\APACrefatitlePlasma environment at the dawn flank of Jupiter’s magnetosphere: Juno arrives at Jupiter Plasma environment at the dawn flank of Jupiter’s magnetosphere: Juno arrives at Jupiter.\BBCQ \APACjournalVolNumPagesGeophys. Res. Lett.444432-4438. {APACrefDOI} 10.1002/2017GL072831 \PrintBackRefs\CurrentBib
- Mingalev \BOthers. (\APACyear2018) \APACinsertmetastarMingalev18{APACrefauthors}Mingalev, O\BPBIV., Malova, H\BPBIV., Mingalev, I\BPBIV., Mel’nik, M\BPBIN., Setsko, P\BPBIV.\BCBL \BBA Zelenyi, L\BPBIM. \APACrefYearMonthDay2018\APACmonth10. \BBOQ\APACrefatitleModel of a Thin Current Sheet in the Earth’s Magnetotail with a Kinetic Description of Magnetized Electrons Model of a Thin Current Sheet in the Earth’s Magnetotail with a Kinetic Description of Magnetized Electrons.\BBCQ \APACjournalVolNumPagesPlasma Physics Reports4410899-919. {APACrefDOI} 10.1134/S1063780X18100082 \PrintBackRefs\CurrentBib
- Mingalev \BOthers. (\APACyear2012) \APACinsertmetastarMingalev12{APACrefauthors}Mingalev, O\BPBIV., Mingalev, I\BPBIV., Mel’nik, M\BPBIN., Artemyev, A\BPBIV., Malova, H\BPBIV., Popov, V\BPBIY.\BDBLZelenyi, L\BPBIM. \APACrefYearMonthDay2012\APACmonth04. \BBOQ\APACrefatitleKinetic models of current sheets with a sheared magnetic field Kinetic models of current sheets with a sheared magnetic field.\BBCQ \APACjournalVolNumPagesPlasma Physics Reports38300-314. {APACrefDOI} 10.1134/S1063780X12030063 \PrintBackRefs\CurrentBib
- Nakamura \BOthers. (\APACyear2008) \APACinsertmetastarNakamura08{APACrefauthors}Nakamura, R., Baumjohann, W., Fujimoto, M., Asano, Y., Runov, A., Owen, C\BPBIJ.\BDBLKhotyaintsev, Y. \APACrefYearMonthDay2008\APACmonth04. \BBOQ\APACrefatitleCluster observations of an ion-scale current sheet in the magnetotail under the presence of a guide field Cluster observations of an ion-scale current sheet in the magnetotail under the presence of a guide field.\BBCQ \APACjournalVolNumPagesJ. Geophys. Res.1137. {APACrefDOI} 10.1029/2007JA012760 \PrintBackRefs\CurrentBib
- Neukirch, Vasko\BCBL \BOthers. (\APACyear2020) \APACinsertmetastarNeukirch20{APACrefauthors}Neukirch, T., Vasko, I\BPBIY., Artemyev, A\BPBIV.\BCBL \BBA Allanson, O. \APACrefYearMonthDay2020\APACmonth03. \BBOQ\APACrefatitleKinetic Models of Tangential Discontinuities in the Solar Wind Kinetic Models of Tangential Discontinuities in the Solar Wind.\BBCQ \APACjournalVolNumPagesApJ891186. {APACrefDOI} 10.3847/1538-4357/ab7234 \PrintBackRefs\CurrentBib
- Neukirch, Wilson\BCBL \BBA Allanson (\APACyear2020) \APACinsertmetastarNeukirch20:jpp{APACrefauthors}Neukirch, T., Wilson, F.\BCBL \BBA Allanson, O. \APACrefYearMonthDay2020\APACmonth06. \BBOQ\APACrefatitleA family of Vlasov─Maxwell equilibrium distribution functions describing a transition from the Harris sheet to the force-free Harris sheet.\BBCQ \APACjournalVolNumPagesJournal of Plasma Physics863825860302. {APACrefDOI} 10.1017/S0022377820000604 \PrintBackRefs\CurrentBib
- Panov \BOthers. (\APACyear2011) \APACinsertmetastarPanov11:magnetopause{APACrefauthors}Panov, E\BPBIV., Artemyev, A\BPBIV., Nakamura, R.\BCBL \BBA Baumjohann, W. \APACrefYearMonthDay2011. \BBOQ\APACrefatitleTwo Types of Tangential Magnetopause Current Sheets: Cluster Observations and Theory Two Types of Tangential Magnetopause Current Sheets: Cluster Observations and Theory.\BBCQ \APACjournalVolNumPagesJ. Geophys. Res.116A12204. {APACrefDOI} 10.1029/2011JA016860 \PrintBackRefs\CurrentBib
- Petrukovich \BOthers. (\APACyear2007) \APACinsertmetastarPetrukovich07{APACrefauthors}Petrukovich, A\BPBIA., Baumjohann, W., Nakamura, R., Runov, A., Balogh, A.\BCBL \BBA Rème, H. \APACrefYearMonthDay2007\APACmonth10. \BBOQ\APACrefatitleThinning and stretching of the plasma sheet Thinning and stretching of the plasma sheet.\BBCQ \APACjournalVolNumPagesJ. Geophys. Res.11210213. {APACrefDOI} 10.1029/2007JA012349 \PrintBackRefs\CurrentBib
- Rich \BOthers. (\APACyear1972) \APACinsertmetastarRich72{APACrefauthors}Rich, F\BPBIJ., Vasyliunas, V\BPBIM.\BCBL \BBA Wolf, R\BPBIA. \APACrefYearMonthDay1972. \BBOQ\APACrefatitleOn the Balance of Stresses in the Plasma Sheet On the Balance of Stresses in the Plasma Sheet.\BBCQ \APACjournalVolNumPagesJ. Geophys. Res.774670-4676. {APACrefDOI} 10.1029/JA077i025p04670 \PrintBackRefs\CurrentBib
- Rong \BOthers. (\APACyear2015) \APACinsertmetastarRong15:venus{APACrefauthors}Rong, Z\BPBIJ., Barabash, S., Stenberg, G., Futaana, Y., Zhang, T\BPBIL., Wan, W\BPBIX.\BDBLZhong, J. \APACrefYearMonthDay2015\APACmonth07. \BBOQ\APACrefatitleThe flapping motion of the Venusian magnetotail: Venus Express observations The flapping motion of the Venusian magnetotail: Venus Express observations.\BBCQ \APACjournalVolNumPagesJ. Geophys. Res.1205593-5602. {APACrefDOI} 10.1002/2015JA021317 \PrintBackRefs\CurrentBib
- Runov \BOthers. (\APACyear2006) \APACinsertmetastarRunov06{APACrefauthors}Runov, A., Sergeev, V\BPBIA., Nakamura, R., Baumjohann, W., Apatenkov, S., Asano, Y.\BDBLBalogh, A. \APACrefYearMonthDay2006\APACmonth03. \BBOQ\APACrefatitleLocal structure of the magnetotail current sheet: 2001 Cluster observations Local structure of the magnetotail current sheet: 2001 Cluster observations.\BBCQ \APACjournalVolNumPagesAnnales Geophysicae24247-262. \PrintBackRefs\CurrentBib
- Saur \BOthers. (\APACyear2018) \APACinsertmetastarSaur18{APACrefauthors}Saur, J., Janser, S., Schreiner, A., Clark, G., Mauk, B\BPBIH., Kollmann, P.\BDBLKotsiaros, S. \APACrefYearMonthDay2018\APACmonth11. \BBOQ\APACrefatitleWave-Particle Interaction of Alfvén Waves in Jupiter’s Magnetosphere: Auroral and Magnetospheric Particle Acceleration Wave-Particle Interaction of Alfvén Waves in Jupiter’s Magnetosphere: Auroral and Magnetospheric Particle Acceleration.\BBCQ \APACjournalVolNumPagesJournal of Geophysical Research (Space Physics)123119560-9573. {APACrefDOI} 10.1029/2018JA025948 \PrintBackRefs\CurrentBib
- Schindler \BBA Birn (\APACyear2002) \APACinsertmetastarSB02{APACrefauthors}Schindler, K.\BCBT \BBA Birn, J. \APACrefYearMonthDay2002\APACmonth08. \BBOQ\APACrefatitleModels of two-dimensional embedded thin current sheets from Vlasov theory Models of two-dimensional embedded thin current sheets from Vlasov theory.\BBCQ \APACjournalVolNumPagesJ. Geophys. Res.1071193. {APACrefDOI} 10.1029/2001JA000304 \PrintBackRefs\CurrentBib
- Schindler \BOthers. (\APACyear2012) \APACinsertmetastarSchindler12{APACrefauthors}Schindler, K., Birn, J.\BCBL \BBA Hesse, M. \APACrefYearMonthDay2012\APACmonth08. \BBOQ\APACrefatitleKinetic model of electric potentials in localized collisionless plasma structures under steady quasi-gyrotropic conditions Kinetic model of electric potentials in localized collisionless plasma structures under steady quasi-gyrotropic conditions.\BBCQ \APACjournalVolNumPagesPhysics of Plasmas198082904. {APACrefDOI} 10.1063/1.4747162 \PrintBackRefs\CurrentBib
- Selesnick \BBA Cohen (\APACyear2009) \APACinsertmetastarSelesnick09{APACrefauthors}Selesnick, R\BPBIS.\BCBT \BBA Cohen, C\BPBIM\BPBIS. \APACrefYearMonthDay2009Jan. \BBOQ\APACrefatitleCharge states of energetic ions in Jupiter’s radiation belt inferred from absorption microsignatures of Io Charge states of energetic ions in Jupiter’s radiation belt inferred from absorption microsignatures of Io.\BBCQ \APACjournalVolNumPagesJournal of Geophysical Research (Space Physics)114A1A01207. {APACrefDOI} 10.1029/2008JA013722 \PrintBackRefs\CurrentBib
- Shkarofsky \BOthers. (\APACyear1966) \APACinsertmetastarBookSJB66{APACrefauthors}Shkarofsky, I\BPBIP., Johnston, T\BPBIW.\BCBL \BBA Bachnynski, M\BPBIP. \APACrefYear1966. \APACrefbtitleThe particle kinetic of plasmas The particle kinetic of plasmas. \APACaddressPublisherAddison-wesley Piblishing company. \PrintBackRefs\CurrentBib
- Sitnov \BOthers. (\APACyear2019) \APACinsertmetastarSitnov19{APACrefauthors}Sitnov, M\BPBII., Birn, J., Ferdousi, B., Gordeev, E., Khotyaintsev, Y., Merkin, V.\BDBLZhou, X. \APACrefYearMonthDay2019Jun. \BBOQ\APACrefatitleExplosive Magnetotail Activity Explosive Magnetotail Activity.\BBCQ \APACjournalVolNumPagesSpace Sci. Rev.215431. {APACrefDOI} 10.1007/s11214-019-0599-5 \PrintBackRefs\CurrentBib
- Sitnov \BBA Merkin (\APACyear2016) \APACinsertmetastarSitnov&Merkin16{APACrefauthors}Sitnov, M\BPBII.\BCBT \BBA Merkin, V\BPBIG. \APACrefYearMonthDay2016\APACmonth08. \BBOQ\APACrefatitleGeneralized magnetotail equilibria: Effects of the dipole field, thin current sheets, and magnetic flux accumulation Generalized magnetotail equilibria: Effects of the dipole field, thin current sheets, and magnetic flux accumulation.\BBCQ \APACjournalVolNumPagesJ. Geophys. Res.1217664-7683. {APACrefDOI} 10.1002/2016JA023001 \PrintBackRefs\CurrentBib
- Sitnov \BOthers. (\APACyear2006) \APACinsertmetastarSitnov06{APACrefauthors}Sitnov, M\BPBII., Swisdak, M., Guzdar, P\BPBIN.\BCBL \BBA Runov, A. \APACrefYearMonthDay2006\APACmonth08. \BBOQ\APACrefatitleStructure and dynamics of a new class of thin current sheets Structure and dynamics of a new class of thin current sheets.\BBCQ \APACjournalVolNumPagesJ. Geophys. Res.1118204. {APACrefDOI} 10.1029/2005JA011517 \PrintBackRefs\CurrentBib
- Sonnerup \BBA Cahill (\APACyear1968) \APACinsertmetastarSonnerup68{APACrefauthors}Sonnerup, B\BPBIU\BPBIÖ.\BCBT \BBA Cahill, L\BPBIJ., Jr. \APACrefYearMonthDay1968\APACmonth03. \BBOQ\APACrefatitleExplorer 12 observations of the magnetopause current layer Explorer 12 observations of the magnetopause current layer.\BBCQ \APACjournalVolNumPagesJ. Geophys. Res.731757. {APACrefDOI} 10.1029/JA073i005p01757 \PrintBackRefs\CurrentBib
- Sonnerup \BBA Su (\APACyear1967) \APACinsertmetastarSonnerup&Su67{APACrefauthors}Sonnerup, B\BPBIU\BPBIÖ.\BCBT \BBA Su, S\BHBIY. \APACrefYearMonthDay1967\APACmonth02. \BBOQ\APACrefatitleLarge Amplitude Whistler Waves in a Hot Collision-Free Plasma Large Amplitude Whistler Waves in a Hot Collision-Free Plasma.\BBCQ \APACjournalVolNumPagesPhysics of Fluids10462-464. {APACrefDOI} 10.1063/1.1762132 \PrintBackRefs\CurrentBib
- Syrovatskii (\APACyear1981) \APACinsertmetastarSyrovatskii81{APACrefauthors}Syrovatskii, S\BPBII. \APACrefYearMonthDay1981. \BBOQ\APACrefatitlePinch sheets and reconnection in astrophysics Pinch sheets and reconnection in astrophysics.\BBCQ \APACjournalVolNumPagesAnnual review of astronomy and astrophysics19163-229. {APACrefDOI} 10.1146/annurev.aa.19.090181.001115 \PrintBackRefs\CurrentBib
- Thomas \BOthers. (\APACyear2004) \APACinsertmetastarThomas04:inbook{APACrefauthors}Thomas, N., Bagenal, F., Hill, T\BPBIW.\BCBL \BBA Wilson, J\BPBIK. \APACrefYearMonthDay2004. \BBOQ\APACrefatitleThe Io neutral clouds and plasma torus The Io neutral clouds and plasma torus.\BBCQ \BIn F. Bagenal, T\BPBIE. Dowling\BCBL \BBA W\BPBIB. McKinnon (\BEDS), \APACrefbtitleJupiter. The Planet, Satellites and Magnetosphere Jupiter. the planet, satellites and magnetosphere (\BVOL 1, \BPG 561-591). \PrintBackRefs\CurrentBib
- Vasko \BOthers. (\APACyear2014) \APACinsertmetastarVasko14:angeo_by{APACrefauthors}Vasko, I\BPBIY., Artemyev, A\BPBIV., Petrukovich, A\BPBIA.\BCBL \BBA Malova, H\BPBIV. \APACrefYearMonthDay2014. \BBOQ\APACrefatitleThin current sheets with strong bell-shape guide field: Cluster observations and models with beams Thin current sheets with strong bell-shape guide field: Cluster observations and models with beams.\BBCQ \APACjournalVolNumPagesAnnales Geophysicae32101349–1360. {APACrefURL} http://www.ann-geophys.net/32/1349/2014/ {APACrefDOI} 10.5194/angeo-32-1349-2014 \PrintBackRefs\CurrentBib
- Vasko \BOthers. (\APACyear2013) \APACinsertmetastarVasko13:pop{APACrefauthors}Vasko, I\BPBIY., Artemyev, A\BPBIV., Popov, V\BPBIY.\BCBL \BBA Malova, H\BPBIV. \APACrefYearMonthDay2013\APACmonth02. \BBOQ\APACrefatitleKinetic models of two-dimensional plane and axially symmetric current sheets: Group theory approach Kinetic models of two-dimensional plane and axially symmetric current sheets: Group theory approach.\BBCQ \APACjournalVolNumPagesPhysics of Plasmas202022110. {APACrefDOI} 10.1063/1.4792263 \PrintBackRefs\CurrentBib
- Waldrop \BOthers. (\APACyear2005) \APACinsertmetastarWaldrop05{APACrefauthors}Waldrop, L\BPBIS., Fritz, T\BPBIA., Kivelson, M\BPBIG., Khurana, K., Krupp, N.\BCBL \BBA Lagg, A. \APACrefYearMonthDay2005\APACmonth05. \BBOQ\APACrefatitleJovian plasma sheet morphology: particle and field observations by the Galileo spacecraft Jovian plasma sheet morphology: particle and field observations by the Galileo spacecraft.\BBCQ \APACjournalVolNumPagesPlanet. Space Sci.53681-692. {APACrefDOI} 10.1016/j.pss.2004.11.003 \PrintBackRefs\CurrentBib
- Walsh \BOthers. (\APACyear2013) \APACinsertmetastarWalsh13{APACrefauthors}Walsh, A\BPBIP., Fazakerley, A\BPBIN., Forsyth, C., Owen, C\BPBIJ., Taylor, M\BPBIG\BPBIG\BPBIT.\BCBL \BBA Rae, I\BPBIJ. \APACrefYearMonthDay2013. \BBOQ\APACrefatitleSources of electron pitch angle anisotropy in the magnetotail plasma sheet Sources of electron pitch angle anisotropy in the magnetotail plasma sheet.\BBCQ \APACjournalVolNumPagesJ. Geophys. Res.1186042–6054. {APACrefDOI} 10.1002/jgra.50553 \PrintBackRefs\CurrentBib
- Watt \BBA Rankin (\APACyear2009) \APACinsertmetastarWatt&Rankin09:prl{APACrefauthors}Watt, C\BPBIE\BPBIJ.\BCBT \BBA Rankin, R. \APACrefYearMonthDay2009\APACmonth01. \BBOQ\APACrefatitleElectron Trapping in Shear Alfvén Waves that Power the Aurora Electron Trapping in Shear Alfvén Waves that Power the Aurora.\BBCQ \APACjournalVolNumPagesPhysical Review Letters1024045002. {APACrefDOI} 10.1103/PhysRevLett.102.045002 \PrintBackRefs\CurrentBib
- Wilson \BOthers. (\APACyear2016) \APACinsertmetastarWilson16:cs{APACrefauthors}Wilson, F., Neukirch, T., Hesse, M., Harrison, M\BPBIG.\BCBL \BBA Stark, C\BPBIR. \APACrefYearMonthDay2016\APACmonth03. \BBOQ\APACrefatitleParticle-in-cell simulations of collisionless magnetic reconnection with a non-uniform guide field Particle-in-cell simulations of collisionless magnetic reconnection with a non-uniform guide field.\BBCQ \APACjournalVolNumPagesPhysics of Plasmas233032302. {APACrefDOI} 10.1063/1.4942939 \PrintBackRefs\CurrentBib
- Xu \BOthers. (\APACyear2018) \APACinsertmetastarXu18:artemis_cs{APACrefauthors}Xu, S., Runov, A., Artemyev, A., Angelopoulos, V.\BCBL \BBA Lu, Q. \APACrefYearMonthDay2018\APACmonth05. \BBOQ\APACrefatitleIntense Cross-Tail Field-Aligned Currents in the Plasma Sheet at Lunar Distances Intense Cross-Tail Field-Aligned Currents in the Plasma Sheet at Lunar Distances.\BBCQ \APACjournalVolNumPagesGeophys. Res. Lett.454610-4617. {APACrefDOI} 10.1029/2018GL077902 \PrintBackRefs\CurrentBib
- P\BPBIH. Yoon \BBA Lui (\APACyear2005) \APACinsertmetastarYL05{APACrefauthors}Yoon, P\BPBIH.\BCBT \BBA Lui, A\BPBIT\BPBIY. \APACrefYearMonthDay2005\APACmonth01. \BBOQ\APACrefatitleA class of exact two-dimensional kinetic current sheet equilibria A class of exact two-dimensional kinetic current sheet equilibria.\BBCQ \APACjournalVolNumPagesJ. Geophys. Res.1101202. {APACrefDOI} 10.1029/2003JA010308 \PrintBackRefs\CurrentBib
- Y\BPBID. Yoon \BOthers. (\APACyear2021) \APACinsertmetastarYoon21:NatCom{APACrefauthors}Yoon, Y\BPBID., Yun, G\BPBIS., Wendel, D\BPBIE.\BCBL \BBA Burch, J\BPBIL. \APACrefYearMonthDay2021\APACmonth01. \BBOQ\APACrefatitleCollisionless relaxation of a disequilibrated current sheet and implications for bifurcated structures Collisionless relaxation of a disequilibrated current sheet and implications for bifurcated structures.\BBCQ \APACjournalVolNumPagesNature Communications123774. {APACrefDOI} 10.1038/s41467-021-24006-x \PrintBackRefs\CurrentBib
- Zelenyi \BOthers. (\APACyear2010) \APACinsertmetastarZelenyi10GRL{APACrefauthors}Zelenyi, L\BPBIM., Artemyev, A\BPBIV.\BCBL \BBA Petrukovich, A\BPBIA. \APACrefYearMonthDay2010\APACmonth03. \BBOQ\APACrefatitleEarthward electric field in the magnetotail: Cluster observations and theoretical estimates Earthward electric field in the magnetotail: Cluster observations and theoretical estimates.\BBCQ \APACjournalVolNumPagesGeophys. Res. Lett.376105. {APACrefDOI} 10.1029/2009GL042099 \PrintBackRefs\CurrentBib
- Zelenyi \BOthers. (\APACyear2011) \APACinsertmetastarZelenyi11PPR{APACrefauthors}Zelenyi, L\BPBIM., Malova, H\BPBIV., Artemyev, A\BPBIV., Popov, V\BPBIY.\BCBL \BBA Petrukovich, A\BPBIA. \APACrefYearMonthDay2011\APACmonth02. \BBOQ\APACrefatitleThin current sheets in collisionless plasma: Equilibrium structure, plasma instabilities, and particle acceleration Thin current sheets in collisionless plasma: Equilibrium structure, plasma instabilities, and particle acceleration.\BBCQ \APACjournalVolNumPagesPlasma Physics Reports37118-160. {APACrefDOI} 10.1134/S1063780X1102005X \PrintBackRefs\CurrentBib
- Zelenyi \BOthers. (\APACyear2022) \APACinsertmetastarZelenyi22{APACrefauthors}Zelenyi, L\BPBIM., Malova, H\BPBIV., Leonenko, M\BPBIV., Grigorenko, E\BPBIE.\BCBL \BBA Popov, V\BPBIY. \APACrefYearMonthDay2022. \BBOQ\APACrefatitleEquilibrium configurations of super-thin current sheets in space plasma: Characteristic scaling of multilayer structures Equilibrium configurations of super-thin current sheets in space plasma: Characteristic scaling of multilayer structures.\BBCQ \APACjournalVolNumPagesJournal of Geophysical Research (Space Physics)127e2022JA030881. {APACrefDOI} 10.1029/2022JA030881 \PrintBackRefs\CurrentBib
- Zelenyi \BOthers. (\APACyear2004) \APACinsertmetastarZelenyi04{APACrefauthors}Zelenyi, L\BPBIM., Malova, H\BPBIV., Popov, V\BPBIY., Delcourt, D.\BCBL \BBA Sharma, A\BPBIS. \APACrefYearMonthDay2004\APACmonth11. \BBOQ\APACrefatitleNonlinear equilibrium structure of thin currents sheets: influence of electron pressure anisotropy Nonlinear equilibrium structure of thin currents sheets: influence of electron pressure anisotropy.\BBCQ \APACjournalVolNumPagesNonlinear Processes in Geophysics11579-587. \PrintBackRefs\CurrentBib
- Zelenyi \BBA Taktakishvili (\APACyear1987) \APACinsertmetastarZelenyi&Taktakishvili87{APACrefauthors}Zelenyi, L\BPBIM.\BCBT \BBA Taktakishvili, A\BPBIL. \APACrefYearMonthDay1987\APACmonth06. \BBOQ\APACrefatitleSpontaneous magnetic reconnection mechanisms in plasma Spontaneous magnetic reconnection mechanisms in plasma.\BBCQ \APACjournalVolNumPagesAstrophysics and Space Science134185-196. {APACrefDOI} 10.1007/BF00636466 \PrintBackRefs\CurrentBib
- Zhang \BOthers. (\APACyear2020) \APACinsertmetastarZhang20:jupiter{APACrefauthors}Zhang, X\BPBIJ., Ma, Q., Artemyev, A\BPBIV., Li, W., Kurth, W\BPBIS., Mauk, B\BPBIH.\BDBLBolton, S\BPBIJ. \APACrefYearMonthDay2020\APACmonth08. \BBOQ\APACrefatitlePlasma Sheet Boundary Layer in Jupiter’s Magnetodisk as Observed by Juno Plasma Sheet Boundary Layer in Jupiter’s Magnetodisk as Observed by Juno.\BBCQ \APACjournalVolNumPagesJournal of Geophysical Research (Space Physics)1258e27957. {APACrefDOI} 10.1029/2020JA027957 \PrintBackRefs\CurrentBib
- Zimbardo (\APACyear1989) \APACinsertmetastarZimbardo89{APACrefauthors}Zimbardo, G. \APACrefYearMonthDay1989\APACmonth07. \BBOQ\APACrefatitleA self-consistent picture of Jupiter’s nightside magnetosphere A self-consistent picture of Jupiter’s nightside magnetosphere.\BBCQ \APACjournalVolNumPagesJ. Geophys. Res.948707-8719. {APACrefDOI} 10.1029/JA094iA07p08707 \PrintBackRefs\CurrentBib